- Details
Small spheres save enzymes for biocatalysis
Plasmas can supply the co-substrate for the biocatalysis of valuable substances, but pity the enzymes. If the latter are attached to small spheres, they work protected and up to 44 times longer.
Some enzymes, such as the one from fungi studied here, are able to produce valuable substances such as the fragrance (R)-1-phenylethanol. To do this, they convert a less expensive substrate using a cosubstrate. A research team from the Department of Biology at Ruhr-Universität Bochum came up with the idea of supplying them with this cosubstrate via a plasma - a crazy idea, as plasmas generally have a destructive effect on biomolecules. However, using several tricks, the researchers led by Prof. Dr. Julia Bandow and Dr. Tim Dirks succeeded. They have now refined one of these tricks and thus improved the process: they attach the enzymes to small balls to hold them to the bottom of the reactor and like this protect them from the harmful influence of the plasma. By choosing the most suitable type of ball, they were able to increase the stability of the enzyme 44-fold. They report in the Journal of the Royal Society Interface from October 25, 2023.
Model enzyme from an edible mushroom
"In plasma-driven biocatalysis, we want to operate enzymes that use hydrogen peroxide to convert a substrate into a more valuable product using technical plasmas," explains Julia Bandow, Head of the Chair of Applied Microbiology. The plasmas - energetically charged gases - produce hydrogen peroxide as well as a variety of reactive species.
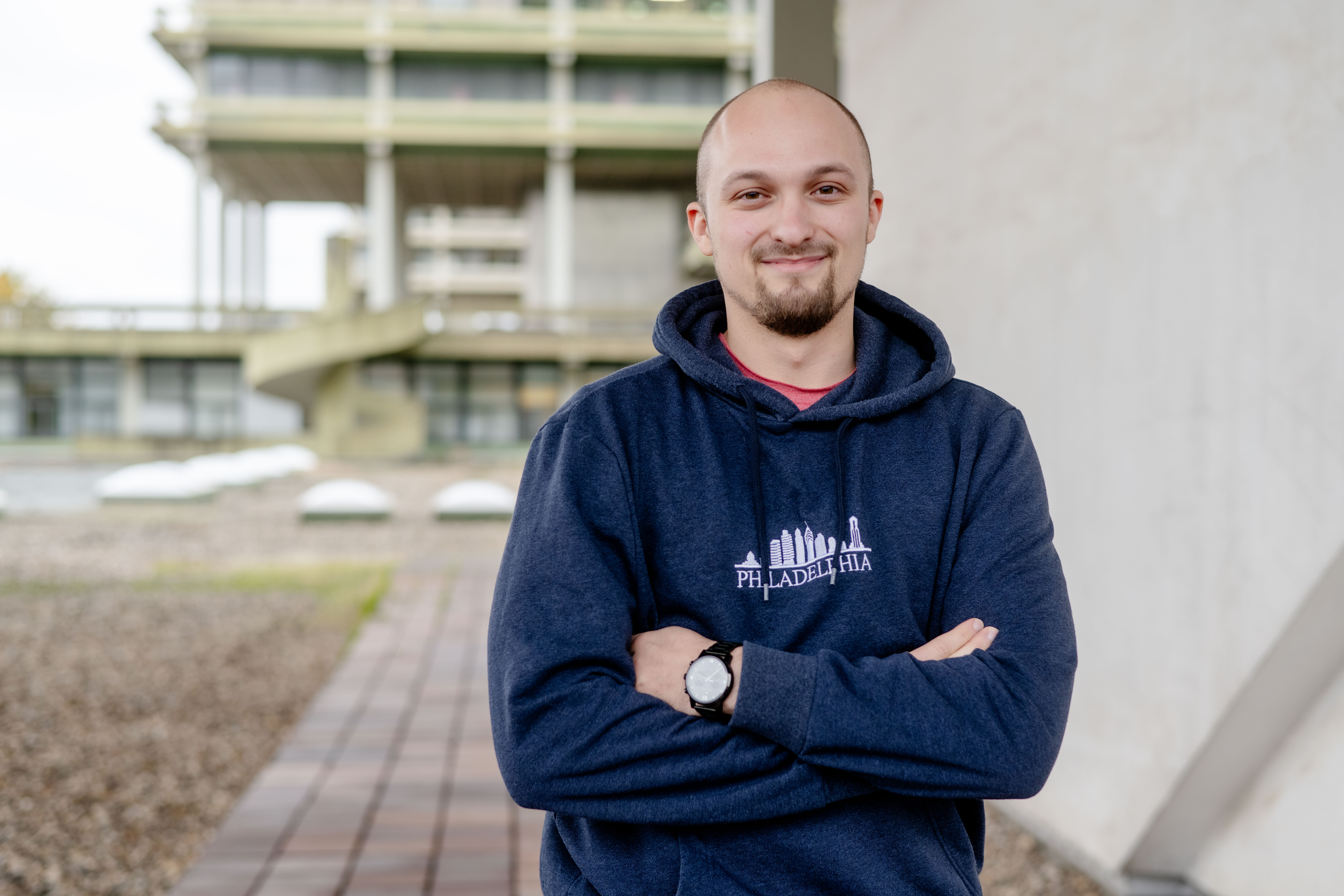
The researchers use the non-specific peroxigenase (AaeUPO) from the edible fungus Agrocybe aegerita as a model enzyme. In initial studies, they were able to show that although plasma-driven biocatalysis works with it, there are also key limitations. "The decisive factor was that the enzymes react sensitively to the plasma treatment and are therefore inactivated within a short period of time," explains Tim Dirks, first author of the current study. "To prevent this, we use the method of enzyme immobilization, i.e. attaching the enzymes to so-called beads: small spheres with a porous surface."
Spheres keep the enzymes at the bottom
Due to gravity, these spheres lie on the bottom of the sample and provide a protective zone between the plasma phase at the top and the enzymes. The research team observed early on that the choice of different immobilization methods also led to different survival rates of enzymes. The aim of the current study was therefore to investigate the effect of different immobilization methods on the plasma stability of enzymes using a larger selection of enzymes.
Five different enzymes were selected, two of which also convert hydrogen peroxide and three of which do not require hydrogen peroxide for their activity. The researchers tested nine different types of beads, some of which had a resin surface and others a silica surface with or without a polymer coating. After immobilization, the enzymes were treated with plasma for up to five minutes. The researchers then compared their residual activity with untreated controls.
The path to new applications
The beads with resin surfaces showed the best results for all five enzymes. "The amino and epoxy-butyl beads performed best," like these," says Tim Dirks. In both cases, the enzymes form a strong, covalent bond with the carrier material, which cannot be dissolved. "This type of immobilization appears to limit the mobility of the enzymes, which makes them less susceptible to plasma-induced inactivation," concludes Tim Dirks. The team extended the plasma treatment times for the most promising candidates to up to one hour and, like this, was able to increase the stability of the enzymes under plasma treatment by up to a factor of 44 through immobilization. "The findings of this study thus pave the way for new applications that aim to combine enzymes with technical plasmas in the future," the researchers like this.
adapted from Maike Drießen, RUB
- Details
Purple flashes on the skin
Cold plasma has an antimicrobial and anti-inflammatory effect. This has also been shown by studies at Ruhr University. Promising applications in medicine and cosmetics are now emerging.
Fine purple lightning flashes through the darkness of the laboratory. It crackles quietly. The flashes are generated inside a 12-by-12-centimeter plasma reactor. After just a few microseconds, the spectacle is over. "This is ionized, particularly high-energy gas or plasma. Most people are familiar with this colourful phenomenon from auroras, which are nothing more than gaseous plasma," says Dr. Friederike Kogelheide from the Chair of Applied Electrodynamics and Plasma Technology at Ruhr-Universität Bochum. "Plasma can be described as the fourth state of matter after solid, liquid and gaseous. There is thermal and non-thermal, i.e. cold plasma," Kogelheide continues like this. The electrical engineer dealt with the latter in her doctoral thesis. It is characterized by a particularly low temperature - at least in comparison to other plasmas, which can reach temperatures of several thousand degrees Celsius. "Cold plasma corresponds approximately to our body temperature, i.e. just over 30 degrees Celsius, and is therefore skin-friendly," explains Kogelheide. Kogelheide has studied the influence of cold plasma on human skin cells, or more precisely, its antimicrobial effect. Her research findings have also led to the founding of her start-up Glim Skin.
For around ten years, researchers in biology, chemistry, medicine and electrical engineering have been investigating the use of cold plasma in medicine. There is great promise here, particularly in the field of wound care and healing. "Studies like this have already shown that molecules produced by plasma, such as nitric oxide, can accelerate wound healing. The beneficial effect of cold plasma is also attributed to the ozone concentration and UV radiation in the plasma," explains Kogelheide. Another advantage of cold plasma is that it corresponds to the body's own temperature and can therefore be applied to human skin both painlessly and without contact. Great hopes are therefore being placed in plasma research.
Fighting resistant bacteria
In order to investigate the antimicrobial effect of cold plasma, the interdisciplinary research team in Bochum led by Kogelheide experimented with spores. "We mainly investigated the effect of plasma on so-called Bacillus subtilis spores. These bacteria are known to be particularly resistant; they can even survive in permafrost. They are therefore considered the gold standard in experiments," explains Kogelheide. The researchers' aim was to specifically reduce and completely kill the spores using cold plasma.
We proceeded in small steps.
– Friederike Kogelheide
"We proceeded in small steps," like this," says Kogelheide. "Our main focus was on the biological substances and building blocks produced by the cold plasma, such as UV radiation, ozone concentration and nitrogen monoxide. How much of this does our plasma produce? Do the spores continue to grow after treatment with plasma? What dose destroys the spores? And what part does the ozone play in this?" Kogelheide's team repeatedly checked and changed the composition of the gas mixture, the treatment time and intensity. Measurements were made using emission and absorption spectroscopy.
The result: it was not the individual components, but only the interaction of ozone, UV radiation and nitrogen monoxide that led to the inactivation of the spores. "The individual components alone had no effect. This is because the substances form a synergy. Another interesting finding was that a natural humidity of around 45 percent relative humidity promoted inactivation," explains Kogelheide.
Lower risk of infection
With their findings, the Bochum researchers have once again provided proof that cold plasma has an antimicrobial effect. They were also able to confirm that plasma produces nitric oxide, which can close wounds. "If we could replace wound healing creams with plasma treatment in the near future, that would be a huge advantage," says Kogelheide. This is because cold plasma can be applied without contact. "In contrast to creams, the risk of infection is significantly lower with plasma applications," explains the scientist.
Plasma flashes in hospital
Anti-inflammatory, wound-healing, antimicrobial: even though plasma research is still in its infancy, promising areas of application are already emerging. "Companies are already waiting in the wings to invest capital in the approval of plasma-based medical products by health insurance companies," observes Kogelheide. At some point, it may be a matter of course that the purple plasma flashes will be flashing through all hospitals.
adapted from Lisa Bischoff (RUB)
- Details
Live monitoring of the inner plasma process
Dr. Moritz Oberberg and two team colleagues are preparing to set up the spin-off “House of Plasma”. The company is launching the Multipole Resonance Probe in various designs. It allows industrial plasmas to be monitored in real time to ensure that they help to consistently apply identical, faultless coatings to surfaces, for example.
Mr. Oberberg, if someone had told you five years ago that you would plan to start a company today, what would you have said?
The idea of setting up a spin-off with measurement technology is not that new. My predecessor, who completed his doctorate in 2015, was already talking about it, but it wasn’t fully developed at the time. Personally, I could always picture myself being self-employed. The timing depends, of course, on the development and the response. Still, I’ve been thinking about self-employment for a while. I have a background in this field and, in addition to my education, I also have the necessary contacts.
The company has not been established yet. How do you keep busy at the moment?
Well, I’m not alone, the three of us share all tasks that come up. We are currently planning industrial tests with a company for our latest designs – we have to travel abroad for this purpose. There’s a lot of red tape. It’s not so easy to take measuring equipment out of the country.
Other than that, I’m busy pushing the development of our products and writing project proposals. I should mention that RUB offers invaluable support with the Worldfactory Startup Center. It has a team of excellent people who help us with everything. At the moment, for example, we are working out how to acquire patents held by RUB. Articles of incorporation are still to be written before we can set up the company, the financial plan is being finalised, and the search for investors is also underway. Before we can sell something, we have to be able to purchase, for which we need capital; the same is true for marketing, premises, equipment and so on. In the current funding project at the university, tests continue in the laboratory and with industrial partners, under difficult conditions due to the pandemic, and the technology is constantly being optimised. This is all going on simultaneously.
What can prospective customers expect from you?
Our prospective clientele might include, for example, a company that coats spectacle lenses. We would check with them what their expectations are and whether our technology offers added value for their company. Can it be used? If yes, then our hardware in combination with the relevant software can provide insights that were not there before.
We compare it to a breakfast egg: I like it when the yolk is still soft but the egg white is hard. However, while it’s cooking, I can only rely on my experience, not really measure what’s going on inside the egg. At most, a peripheral measurement of the temperature on the shell would be possible, but not inside the egg. What we do now, however, is supply the data straight from the egg.
adapted from Meike Drießen (RUB)
- Details
The biggest challenge is people's minds
Research data management - that sounds primarily technical. But a social component is also crucial, as plasma researcher Achim von Keudell knows to report.
At first glance, storing data in a structured and well-documented manner does not seem to be a challenge. But if you look at this task in detail, there are some hurdles to overcome. The team in Collaborative Research Center (CRC) 1316 "Transient Atmospheric Pressure Plasmas" has taken up the cause. SFB spokesman Prof. Dr. Achim von Keudell tells us in an interview why there were some thick boards to drill.
Professor von Keudell, why is research data management a challenge in your field?
Fifty percent of research in physics is done in large-scale research projects, such as in astrophysics or nuclear physics, where there are standards for data storage. The rest has been held in small laboratories. There, it is common for each group to use its own programs for data analysis or for creating graphics, or to use its own tools for data storage. In addition, the data from different experiments are very heterogeneous. The biggest challenge, however, is people's minds.
Why?
At first, many don't see the added value of research data management. They are worried that others could steal their data if it is handled too transparently. I have done a lot of promotion for the topic at conferences of the German Physical Society - and I was surprised how much convincing has to be done and what kind of resistance you have to fight against. It's a bit like telling a teenager "Clean your room" and getting the answer "Why? I can find everything."
Younger researchers are often open to research data management. Others often don't see the benefits at first, because they've gotten by for a long time without a standardized form of data storage and documentation. In the meantime, however, research funding organizations and scientific journals are demanding transparency.
Yes, the question of whether you have to make data accessible has not existed for one or two years. The German Research Foundation supports research data management, and you can no longer publish in many journals without publishing your data in a repository in parallel. Nevertheless, we still have a dormant treasure here that needs to be lifted.This is sustainable, especially when you consider that this data was produced with taxpayers' money.
In what way?
It's not just about publishing the data somehow, but doing it like this so that the next generation of researchers can continue to work with it. That's sustainable, especially considering that this data was produced with taxpayers' money.
In our Collaborative Research Center, for example, we have worked with colleagues to develop standards for metadata: When I perform a measurement, it is not only the results that are interesting. In order for someone to be able to reproduce the data later, it may also be relevant to know what the temperature was in the lab and which measuring device was used to make the recording. For various experiments, we discussed which metadata needed to be documented, and in the SFB we now use a common repository to store the data with uniform standards.
How easy was that to do?
We were able to build on good preliminary work from our colleagues at the Leibniz Institute INP in Greifswald, who had already given a lot of thought to the topic and recommended a particular open source software solution. However, as mentioned before, the data generated in different experiments is very different. Pouring that into a larger format that everyone is happy with is not easy. But we now have a system that about 50 people from different areas of plasma research - from engineered plasmas to biotechnology - are using, and we are continuously developing it.
In the meantime, something is changing in people's minds!
And how does the broad plasma community feel about the topic in the meantime?
In the meantime, we know that it is particularly convincing to present successful individual examples. If you only discuss at the level of FAIR principles, it remains too abstract. Concrete application cases help to convince people. And in the meantime, something is changing in people's minds!
Many now see the advantages. If we store data in a well-documented and accessible way, researchers can get access to a wide variety of measurements and perform cross-cutting analyses. This has not been done much before, but it enables completely new insights. It has crazy potential.
If you get involved early, you have the chance to help shape it!
What do you tell colleagues who are still skeptical?
That it's better to participate wholeheartedly from the start than to have to realize after ten years of resistance that there's no way around it anyway and that standards have been established that don't suit you. If you join in early, you have the chance to help shape things!
In the meantime, we know that it is particularly convincing to present successful individual examples. If you only discuss at the level of FAIR principles, it remains too abstract. Concrete application cases help to convince people. And in the meantime, something is changing in people's minds!
Many now see the advantages. If we store data in a well-documented and accessible way, researchers can get access to a wide variety of measurements and perform cross-cutting analyses. This has not been done much before, but it enables completely new insights. It has crazy potential.
If you get involved early, you have the chance to help shape it!
What do you tell colleagues who are still skeptical?
That it's better to participate wholeheartedly from the start than to have to realize after ten years of resistance that there's no way around it anyway and that standards have been established that don't suit you. If you join in early, you have the chance to help shape things!
adapted from Julia Weiler (RUB)
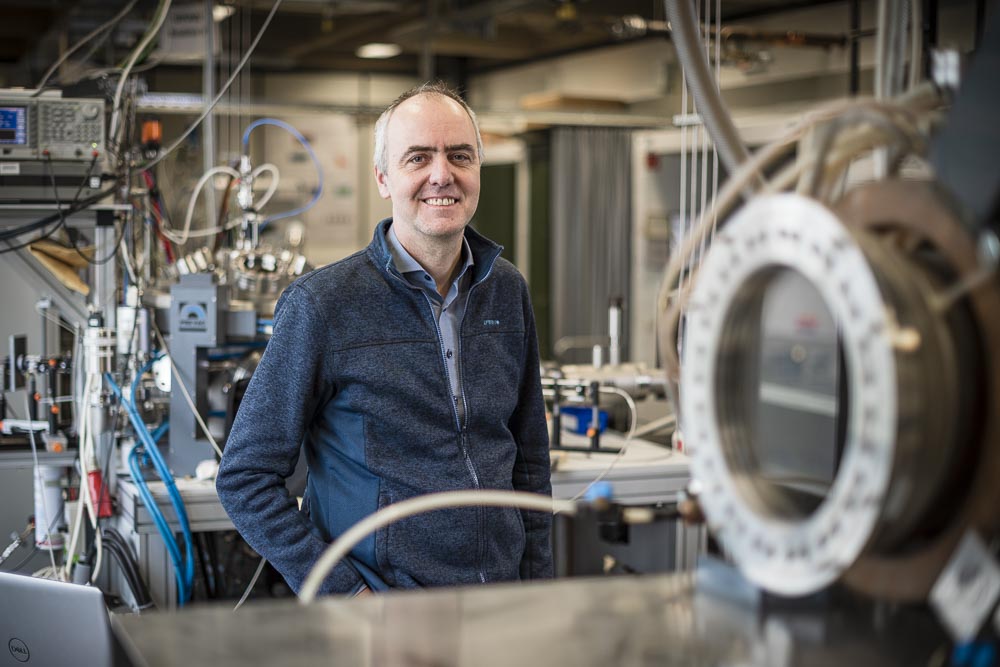
- Details
Young scientists benefit from international exchange
To control plasma-produced carbon monoxide and use its anti-inflammatory effects on wound healing is the aim of a cooperation between Professor Judith Golda from Ruhr University Bochum, Germany, and Professor Claire Douat from Université d’Orléans, France. In six mutual visits their groups experimented together, financed by the German Academic Exchange Service and by Campus France.
Ms. Golda, what would you like to achieve?
Judith Golda: Today plasma is not yet a well-understood, efficient and easy tool in medicine, I would say. We hope that in the future doctors can simply use a plasma-source to cure diseases of the skin.
For which medical applications is plasma already used?
Golda: A few hospitals in Germany are utilizing it for wound healing within clinical studies. Moreover, there are surveys for cancer therapy, sterilisation…
Claire Douat: … the treatment of teeth, skin diseases like psoriasis and acne, for cosmetics and blood coagulation, to avoid or reduce a bleeding.
How exactly does plasma work in wound healing?
Golda: There are different effects. One of them is sterilisation, another blood coagulation. Plasma can also increase the blood flow in the wound. And it can work anti-inflammatory by producing reactive species that resemble natural messenger substances.
In what way do your teams complement each other?
Golda: In France, the plasma source is a little bit different from ours. It produces more charged species in the vicinity of the treated substrate and a different mixture of chemical species, for example. On the one hand, we compare the plasma sources, on the other hand we exchange complementary diagnostics: In Orléans they use optical sensors for measuring the gas density – such as the one for carbon monoxide, in Bochum we employ mass spectrometry.
Why is it difficult to prove the impact of a single plasma component?
Douat: In a plasma there are many chemical reactions happening simultaneously. We have the electric field, reactive oxygen species and the light. So, it is not only difficult to explore but also to control it. At some concentrations those reactive species are beneficial, but at higher ones they can become toxic. In sterilisation or cancer treatment we want this toxicity, but in wound healing we don’t.
Now we have a lot of science to discuss.
– Claire Douat
What is characteristic of the cold atmospheric pressure plasma you use for the treatment of biological substrates?
Golda: Unlike other plasmas it stays cold enough not to harm the skin. The idea is to channel the energy we put in the plasma into the electrons to heat them. In this condition they are able to dissociate molecules and create reactive species while the gas remains close to room temperature. That is this non equilibrium character that everybody is talking of.
Which substrates do you use for your experiments?
Douat: Right now, we are utilizing bacteria. The next step will be on cells and afterwards on animals. But before, we need to make sure that the treatment is safe, for example that the electric current through the biological target is not too high.
What could you find out until now?
Douat: We performed electrical measurement and determined the concentration of some species in the different plasma-sources. For that we have one from Bochum in Orléans and they have one from us in Bochum. In the end, we treated bacteria to see the effects of the two plasma-sources: the bacteria didn’t behave in the same way when treated with diverse plasmas. Which is nice, because now we have a lot of science to discuss and are writing a paper about it.
Internationally, Ruhr University is a very well-known place for plasma research, with leading plasma and modelling experimental physicists.
– Claire Douat
Ms. Douat, what is your impression of Ruhr University?
Douat: I really like being in Bochum. Every time I come here, it’s easy. There is all this infrastructure with the hotel and everything I need in walking distance to university – including supermarkets and even a swimming pool.
From my point of view, when we are every day in the same place, we see things not the way we see them as a visitor. In Bochum they are well organised and there is always room for ease. Also internationally, Ruhr University is a very well-known place for plasma research, with leading plasma and modelling experimental physicists.
It’s fine to be here and to discuss with them. In addition, they have a big pack of experimental setup. I would not say everything, but a lot. When we want to test something, there is always a way to make it possible.
Golda: Also, the mensa has vegan and vegetarian options. One of the French students is vegetarian …
Douat: … and the view is great!
Sometimes it’s really inspiring to be in a new environment like another lab or university to get new ideas.
– Judith Golda
What are the advantages of your cooperation?
Golda: It’s a lot of fun. I like co-operating with Claire and her group. When you are working on a topic on your own, from time to time you get stuck on your ideas. In a cooperation we can discuss the issues and develop new ideas together. Sometimes it’s really inspiring to be in a new environment like another lab or university to get new ideas. In addition, Claire and her team have some expertise we don’t have.
Douat: It’s always fine to discuss and see another point of view. The students also work together and learn from each other. That’s a very good experience for them.
Golda: You really see how they grow. The first time the students go to France they are rather shy and seem kind of lost. The second time it’s like they feel at home there. The relationships between them change a lot and it’s awesome to watch them meeting at the weekend and spending free time together.
Douat: When we come to Bochum or our colleagues visit us in Orléans, it’s a bit like holidays, at least for Judith and me. Not in the sense that we wouldn’t work. We work a lot when we travel. But in that time, we have only one task to do, so we can focus on it. At home, we are busy with so many different things every day. Right now, we enjoy just to be at the laboratory, the whole day working on one project.
adapted from Carina Huber (RUB)
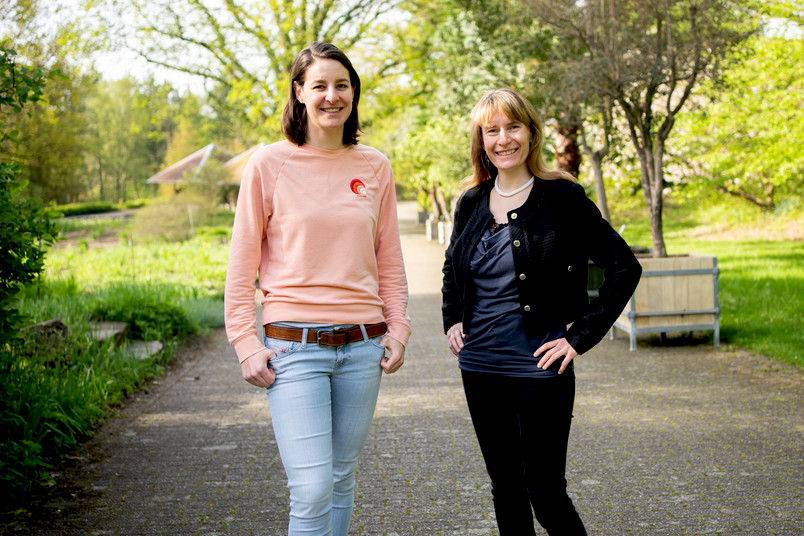
- Details
A sieve for molecules
Researchers have long tried to use graphene, which is made of carbon, as a kind of sieve. But it has no pores. Now a team has found an alternative material that provides the holes on its own.
Researchers from Bielefeld, Bochum and Yale have succeeded in producing a layer of two-dimensional silicon dioxide. This contains natural pores and can therefore be used like a sieve for molecules and ions. Scientists have been searching for such materials for some time, as they could help desalinate seawater or be used in new types of fuel cells. The team describes the fabrication process in the journal Nano Letters, published online Jan. 19, 2022. The teams led by Dr. Petr Dementyev of Bielefeld University, Prof. Dr. Anjana Devi of Ruhr University Bochum and Prof. Dr. Eric Altman of Yale University collaborated on the work.
When two-dimensional materials are pierced with high precision, they can be used to screen out specific ions or molecules. Researchers have repeatedly tried to use graphene, a material made of carbon atoms, for this purpose. Since it has no natural pores, they have to be inserted artificially. But it is difficult to create holes of a defined size in graphene without permanently damaging the material, which breaks easily. This is because it loses too much stability due to the perforation. Consequently, an alternative had to be found. In the current work, the research team took advantage of the fact that the crystal lattice of two-dimensional silicon dioxide naturally has openings. They showed that these openings can be used to separate certain gases.
"Silicon dioxide naturally has a very high density of tiny pores that could not be created in artificial membranes," says Petr Dementyev of the Bielefeld-based Physics of Supramolecular Systems and Surfaces group. "Unlike graphene, the pores are all nearly the same size. And there are so incredibly many that the material behaves like a fine-mesh sieve for molecules."
2D silica has been known since 2010. However, its production was very expensive and only possible on a small scale. The researchers from Bochum, Bielefeld and Yale brought together expertise from materials chemistry, chemical engineering and chemical physics to devise a new manufacturing process. They used what is known as atomic layer deposition to deposit a single layer of silicon dioxide on a gold surface. Using a high-pressure process, the researchers transferred the layer to its two-dimensional form and then characterized it in detail spectroscopically and microscopically. They then studied the gas flow through the 2D membrane in a vacuum chamber.
While evaporated water and evaporated alcohol were able to pass through the silica layer, the gases nitrogen and oxygen were retained. "Materials like this with selective permeability are in high demand in industry," says Anjana Devi. However, before the 2D silica can be used in practice, it is important to evaluate exactly how many different molecules can attach to or penetrate the surface of the material.
"We expect our results to be important for materials science worldwide," sums up Anjana Devi of the Inorganic Materials Chemistry group in Bochum. "Such 2D membranes could help at the forefront of sustainable development, for example in the field of energy conversion or storage."
adapted from RUB, Julia Weiler
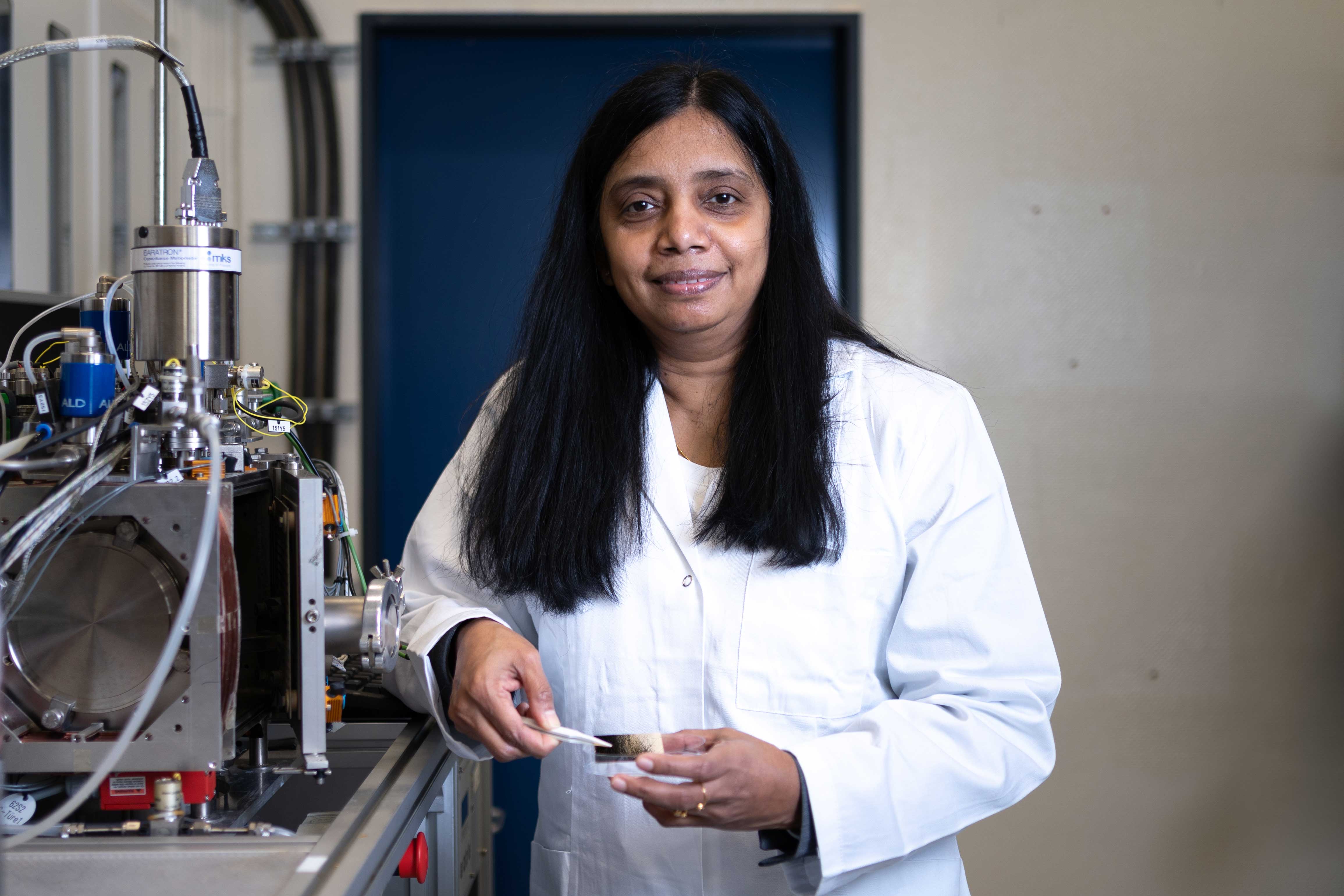
- Details
Applied plasma research in science magazine Rubin
Our everyday lives are virtually inconceivable without plasmas. A special edition of Rubin provides insights into their numerous areas of application.
Plasmas help heal wounds, clean up exhaust gases efficiently and extend the shelf life of drinks in PET bottles. They affect our everyday lives in many ways, often without us noticing. The various applications of plasmas are featured in a special edition of Rubin, the RUB’s science magazine. The issue, published on 1 September 2021, gives insights into the activities of two Collaborative Research Centres (SFB/CRC): SFB/TR 87 “Pulsed high power plasmas for the synthesis of nanostructural functional layers” has been operating at RUB since 2010, and CRC 1316 “Transient atmospheric pressure plasmas: from plasmas to liquids to solids” since 2018. Both centres present their research in Rubin.
by Julia Weiler (RUB)
- Details
Plasmas facilitate the production of small structures
Martin Hoffmann explains why plasma processes are essential for microsystem technology and which opportunities they offer for eco-friendly manufacturing methods.
Plasmas are the tool of choice for microsystem technology. As electronic chips are getting smaller and smaller, structures can only be realized with dry, plasma-assisted processes. Wet chemical processes no longer work in these dimensions. During drying, small, movable structures are glued together by surface tension, just as two sheets of glass with a very thin film of water between them can hardly be separated. New materials such as glasses or 2D semiconductors require new processes for deposition and structuring.
The key is specially adapted plasmas. In addition, we need to significantly advance the methods for measuring the internal parameters of a plasma and the relevant process control in real time. This is the only way we can also achieve reproducible results in batch production. In addition, plasma processes offer resource-saving, environmentally friendly manufacturing methods with minimal material input – even when coating with new types of materials. This future begins now, with the construction of research facilities at the boundary between basic and applied research and cross-disciplinary cooperation to enable the use of innovative materials.
- Details
Plasma generators control catalytic processes
In ten years, researchers will have understood the interactions between catalysts, which determine the speed of chemical reactions, and plasmas. This will facilitate the excitation of the plasma at atmospheric pressure in such a way that its properties accelerate the reactions on the catalyst surface in a controlled manner. As a result, chemical engineers will not only increase the turnover of the starting materials, but also the percentage of these materials that will be converted into the required product.
Therefore, the vision is that plasma generators will control catalytic processes. New compact plasma catalyst modules will be created, through which large gas flows can pass with little pressure drop. This will enable exhaust gas streams to be purified and other important industrial reactions to be carried out. In order for the modules to work in a resource-saving way, researchers still have to boost their energy efficiency. In future, catalysis, plasma and reaction engineering experts will work hand in hand to develop plasma catalyst modules. Computer-aided plasma, velocity and flow simulations will help to optimise them.
by Martin Muhler
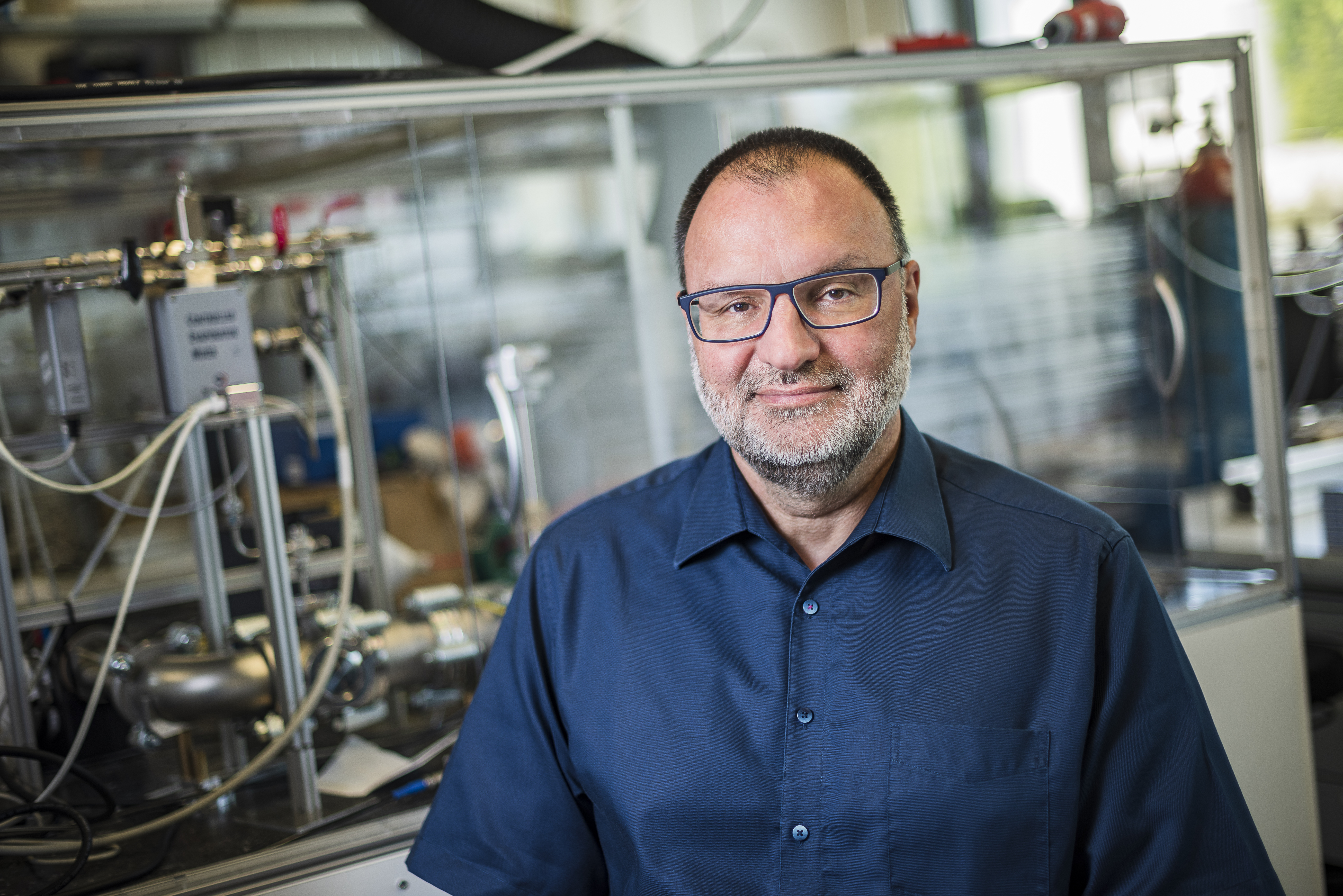
- Details
How cola still tingles after a year
When you add energy to gases or gas mixtures, a plasma can be created, and inside it things go haywire: atoms turn into ions, free electrons whiz through space and collide with everything, some ingredients decay, other substances form anew. Depending on what is added to the starting material, plasmas can therefore be used to produce larger compounds. Hydrocarbons and silicon hydrogens are turned into long chains of molecules called polymers.
“If you want to etch with plasmas that tend to form polymers, it’s bad because the nanoparticles that form are a hindrance,” explains Professor Peter Awakowicz, holder of the Chair of General Electrical Engineering and Plasma Technology at RUB. But his team has taken advantage of the situation. If polymers are specifically made to form and deposit on the surfaces surrounding the plasma, they can be coated in a targeted manner. Thanks to this so-called Plasma Enhanced Chemical Vapor Deposition, or PECVD for short, it is possible, for example, to apply ultra-thin, gas-tight coatings to the inside of PET bottles, ensuring that the contents last longer, or to protect organic light-emitting diodes (OLEDs) from moisture so that the TV screens work for a long time. This and much more is only possible because the plasmas are cold and thus do not damage the PET bottle or other surfaces to be coated with heat. Only the fast electrons in the plasma are hot, and they do not damage the surfaces.
Making milk and medicines last longer
The glass-like coating of the plastic, which is only 20 to 30 nanometres thin, ensures that 10 to 100 times less gas escapes through the bottle. This extends the shelf life of a soda pop from the previous four weeks to about a year. The method is also of interest for the packaging of milk and other foods, as well as medicines and even microelectronic components.
“This type of coating is also environmentally friendly, because the tiny amount of material can simply be neglected during recycling,” explains Dr. Marc Böke from the Experimental Physics II department at RUB. Composite materials made of plastic and aluminum, such as Tetrapaks, are far more difficult to recycle because it is very difficult to separate the components.
Other applications of the PECVD method can be, for example, the coating of implants that grow into the bone better than conventional ones. There are also many microelectronic applications. For example, transistors can be deposited with ultrathin silicon dioxide films in plasma.
Oxygen tips the scales
The challenge lies in controlling the formation of the layers. “The layers should not only be ultra-thin, but also absolutely dense, gap-free and uniform,” explains Marc Böke. The adjusting screws for this are manifold. For one thing, it depends on the gas mixture. Atomic Oxygen is a particularly important player. Its proportion can be used to control, among other things, whether other additives evaporated into the plasma form inorganic layers, such as the glass-like silicon dioxide, or organic layers that have other interesting properties, such as giving surfaces greater biocompatibility or enabling gas separation.
The pressure at which the plasma is operated is also significant. Higher pressures and corresponding gases result in the coating of surfaces, while lower pressures are more likely to result in etching processes, which are central to all microelectronics (from cell phones to modern cars). Similarly, the geometry of the reactor and the choice of energy source influence what happens in the plasma and how it affects the surrounding surfaces. For example, an appropriate plasma can be ignited by microwaves, but also by inductively or capacitively coupled radio frequency. “In general, different sizes of plasma reactor are possible, up to the huge dimensions needed to coat entire window panes for high-rise buildings,” says Peter Awakowicz. These coatings serve to reflect infrared radiation that would otherwise cause it to get as hot behind them as in a greenhouse when the sun shines. But you can still see through it. With the sputtering of thin metal layers on foils used for this purpose, it is also possible to work in a feed-through process and thus coat many square metres.
Measurement techniques had to be developed
Only after the basic mechanisms of high-power pulsed sputtering (HiPIMS) and PECVD had been measured and understood in the first phase of the Collaborative Research Center SFB/TR 87 the research teams could get down to the business of implementing such large-area coatings. “We had to develop the appropriate measurement techniques in some cases,” Awakowicz recounts. “If you simply hold a measuring probe in the plasma, it may become coated itself and may lose its function,” he gives an example.
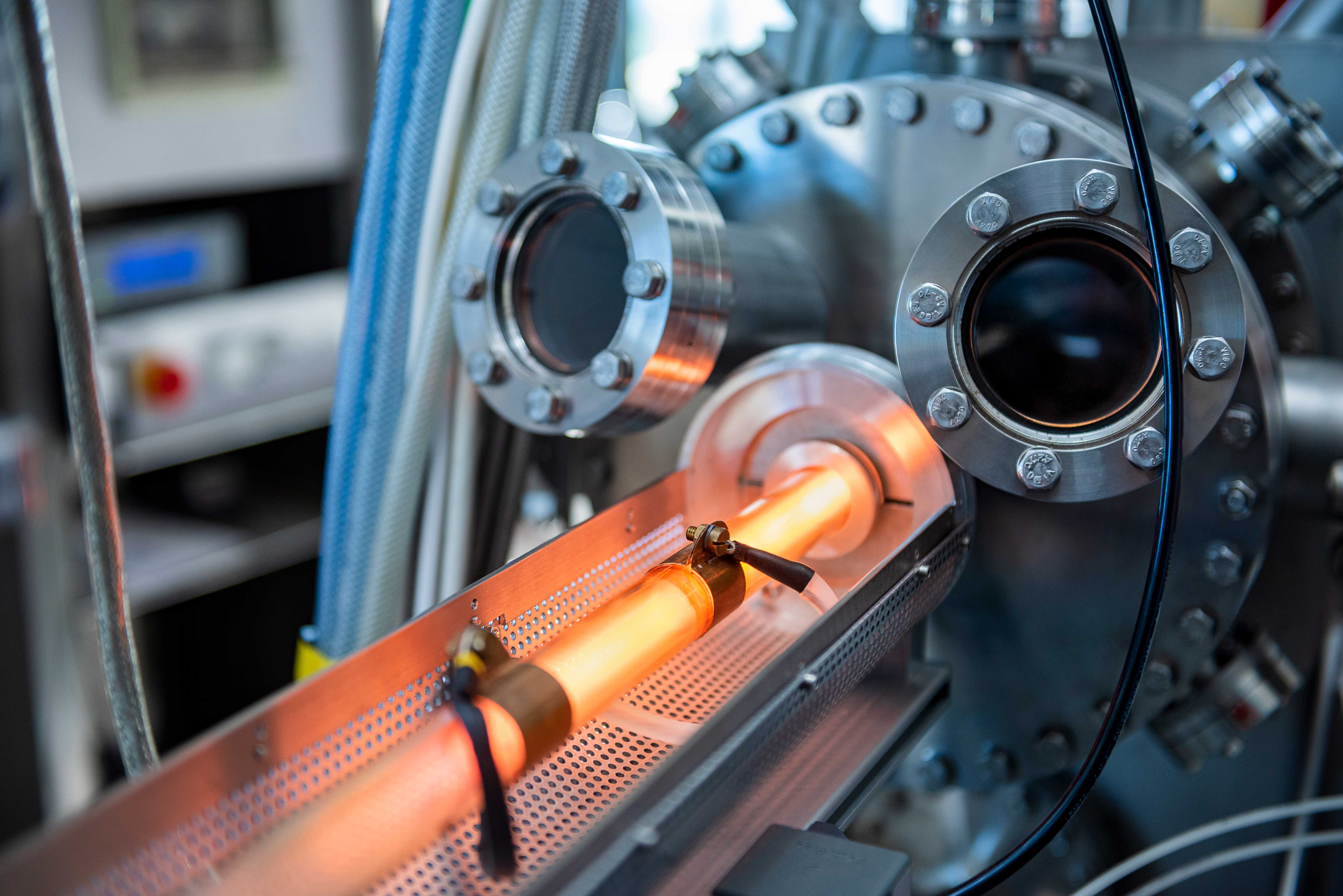
The researchers have gradually been able to fathom and perfect many aspects of the possible processes. For example, PET bottles are cleaned and activated before coating, also by means of plasma. But here, too, the surface of the bottle changes, which in turn influences the subsequent coating. Measurements of the particle flows during cleaning revealed what happens in the process: is wettability increased? And if so, how? Does the surface energy change? At what point in the treatment does the surface become roughened? “If the surface is too rough, you can no longer cover it evenly with an ultra-thin coating,” explains Marc Böke. If all these aspects are taken into account during cleaning and the process is run optimally, this has a considerable influence on the success of the subsequent coating: “We were able to increase the impermeability, which was initially a factor of 100 (depending on the substrate material), to a factor of 500 through the correct setting of the previous cleaning,” says Peter Awakowicz.
Keeping plasticizers away from food
Detailed knowledge of the processes in the plasma and the resulting coatings now also make it possible to coat stretchable films with gas-tight thin films. Thanks to an intervening buffer layer, Marc Böke’s team was able to increase the tolerance of the layer to the stretching of the film from originally about three to about six percent. This application is also of interest to the food industry, for example, as the dense coating prevents ingredients from the film, such as the dreaded plasticizers, from penetrating the food.
The latest application, which is currently being worked on, makes a virtue out of necessity: if one actually wishes for layers that are as dense and defect-free as possible, defects such as tiny pores in the coating are almost impossible to avoid. They allow the research teams to use plasma coating to develop non-swelling filter membranes that exhibit previously unknown properties. They can desalinate water or separate gases from each other, such as oxygen from CO2. “Normally, the more selective a membrane is, the lower its transmission, i.e. the more inefficient the process,” explains Marc Böke. “With plasma coating, however, we can control pore formation so that selectivity no longer comes at the expense of transmission or efficiency.” The researchers of the SFB/TR87 can simulate and tailor the polar properties of the membrane. This makes it easier for certain molecules to pass through the membrane. “Water molecules, for example, are made to give up their actual angle, practically flattening out and thus sliding through the pore,” Peter Awakowicz describes. “You couldn’t target something like that before.”
adapted from Meike Drießen (RUB)
- Details
Plasmas aid in wound healing, cancer therapy and pollutant degradation
Reactive oxygen and nitrogen species (RONS) have different functions in biological contexts: At low concentrations they act as signalling substances, for example in wound healing. At high concentrations they destroy biomolecules, an effect immune cells use to kill pathogens. Non-equilibrium plasmas, in which the electrons have high temperatures but the gas temperature remains low, can produce such RONS without heating the treated samples. These non-thermal plasmas are already being used for sterilisation purposes. Their therapeutic use in wound treatment and cancer therapy is currently being explored.
In ten years, we will understand in more detail the mechanisms underlying the generation of the different reactive particles in plasma as well as their biological effects. Based on this knowledge, plasma reactors can then be designed that provide RONS at concentrations and with mixing ratios required for specific applications – such as reactions catalysed by enzymes that utilise plasma-generated species or the degradation of pollutants through the joint action of plasmas and microbes.
by Julia Bandow (RUB)
- Details
What exactly happens at the interface between plasma and surface
Plasmas have been used in industry for decades with great success. However, in many cases their application is based on trial and error. A deeper understanding of the processes that take place in the excited gases, sometimes in a very short time and on tiny length scales, has been largely lacking until now. Researchers from Bochum and Ulm are venturing into this new territory together in the frame of the Collaborative Research Centre 1316 “Transient Atmospheric Pressure Plasmas: From Plasmas to Liquids to Solids”.
Their fields of work are complementary: Professor Thomas Mussenbrock, who holds the Chair of Applied Electrodynamics and Plasma Technology at RUB, focuses on the plasma as a whole, whereas Professor Timo Jacob, head of the Institute of Electrochemistry at Ulm University, specialises in atomic processes. “We calculate processes at different ends of the time and length scales and try to fill the gap in between,” as Mussenbrock recaps.
Complicated molecules
Timo Jacob is the expert for interfaces between plasmas and their environment. This interface can be between plasma and solid, but also between plasma and liquid. “If we want to use plasmas to coat a surface or to change its structure, we have to understand the interaction between the plasma species and the surface on an atomic scale,” he explains. This process seems simple and predictable. But his team goes into much more detail, because complicated molecules can also form in plasmas, which are present in specific excited states. They can then change both the properties and the structure of catalytic surfaces or even actively impact reactions at the interface directly. Until now, such details had been little understood. “We had to develop the tools for this first,” says Jacob.
He and his team established methods that can describe how the plasma changes the molecules during a reaction. The influence of the molecules, for example, can result in an effect on the interface that becomes meta-stable but of particular interest for catalytic reactions.
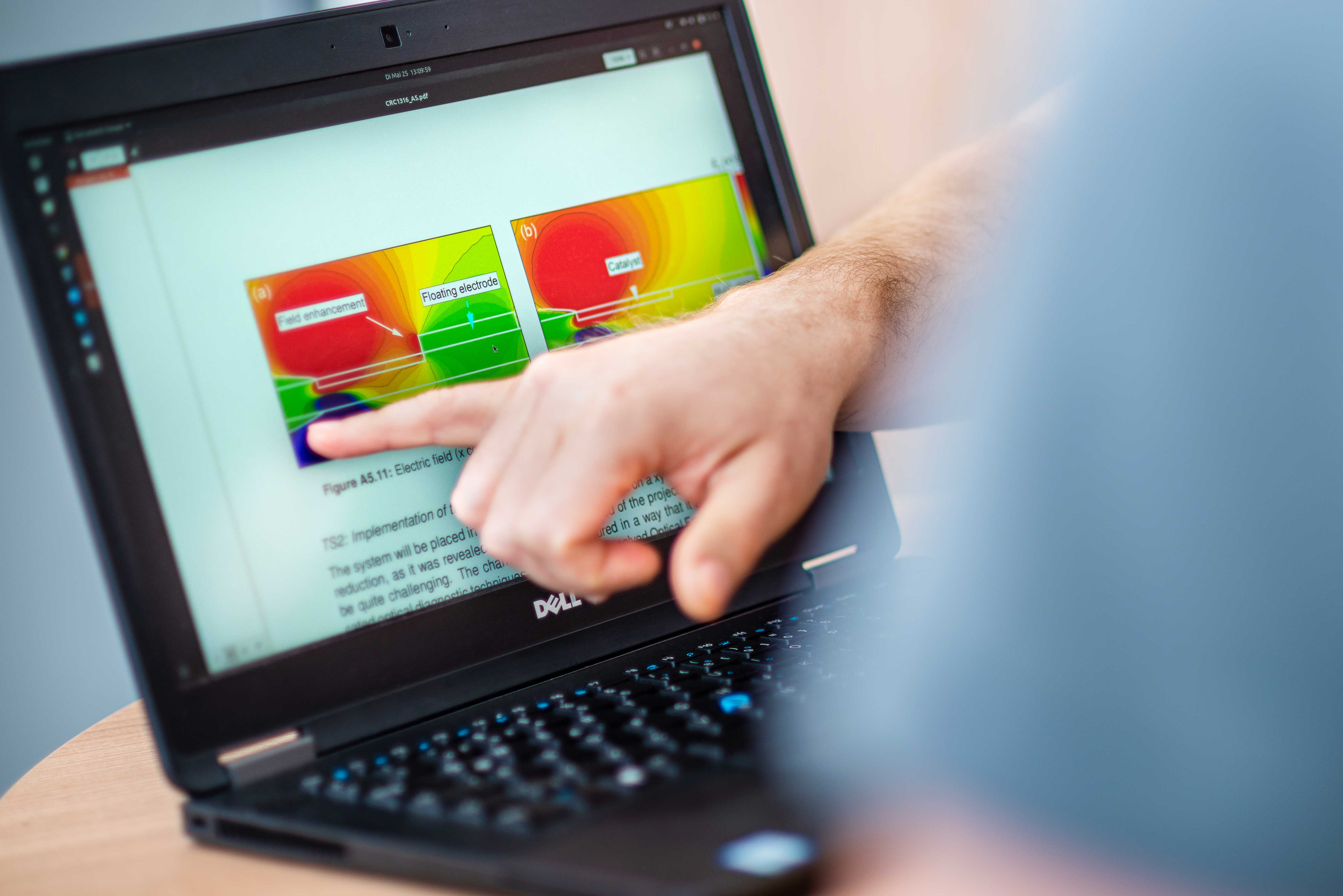
The effort usually increases cubically
The processes calculated in this manner take place on the Ångström or nanometre scale within a tiny fraction of a second. The fewer effects the researchers have to calculate simultaneously, the more accurate the simulation can become. “Our highly accurate calculations involve a maximum of 300 to 400 atoms; using semi-empirical dynamic simulations even several 100,000 atoms are possible,” explains the researcher. “We sometimes use specifically acquired computing clusters or mainframes at high-performance computing centres for this purpose.” The effort usually increases cubically: i.e. if twice as many atoms are to be included in the calculation, the computational effort increases eightfold.
The fundamental insights thus gained should help, for example, to make plasmas usable for new applications – most importantly, catalytic processes. The researchers concentrate on both the reduction of carbon dioxide (CO2) and the decomposition of relatively simple hydrocarbons as a model, using n-butane as an indicator.
Data is extremely scarce
It is of course not enough to consider only a few hundred atoms. This is where the research of Thomas Mussenbrock’s team completes the overall picture. His length scale is a billion times larger than Jacob’s. Quantum mechanical effects that are important at the atomic level no longer need to be explicitly considered in this case. The idea is to map plasma dynamics and plasma chemistry. “We look at the plasma based on classical physics.” The methods for this are known and well understood, but the needed data is extremely scarce.
A major challenge is that industrial processes with plasmas should take place at atmospheric pressure, if possible, and preferably at air pressure. “At this relatively high pressure, many interactions come into play,” explains Mussenbrock. What reacts how strongly with which molecules and atoms? Based on the results of Timo Jacob’s calculations, his team scales up the simulation. The results can be matched with experiments – deviations must be understood and explained.
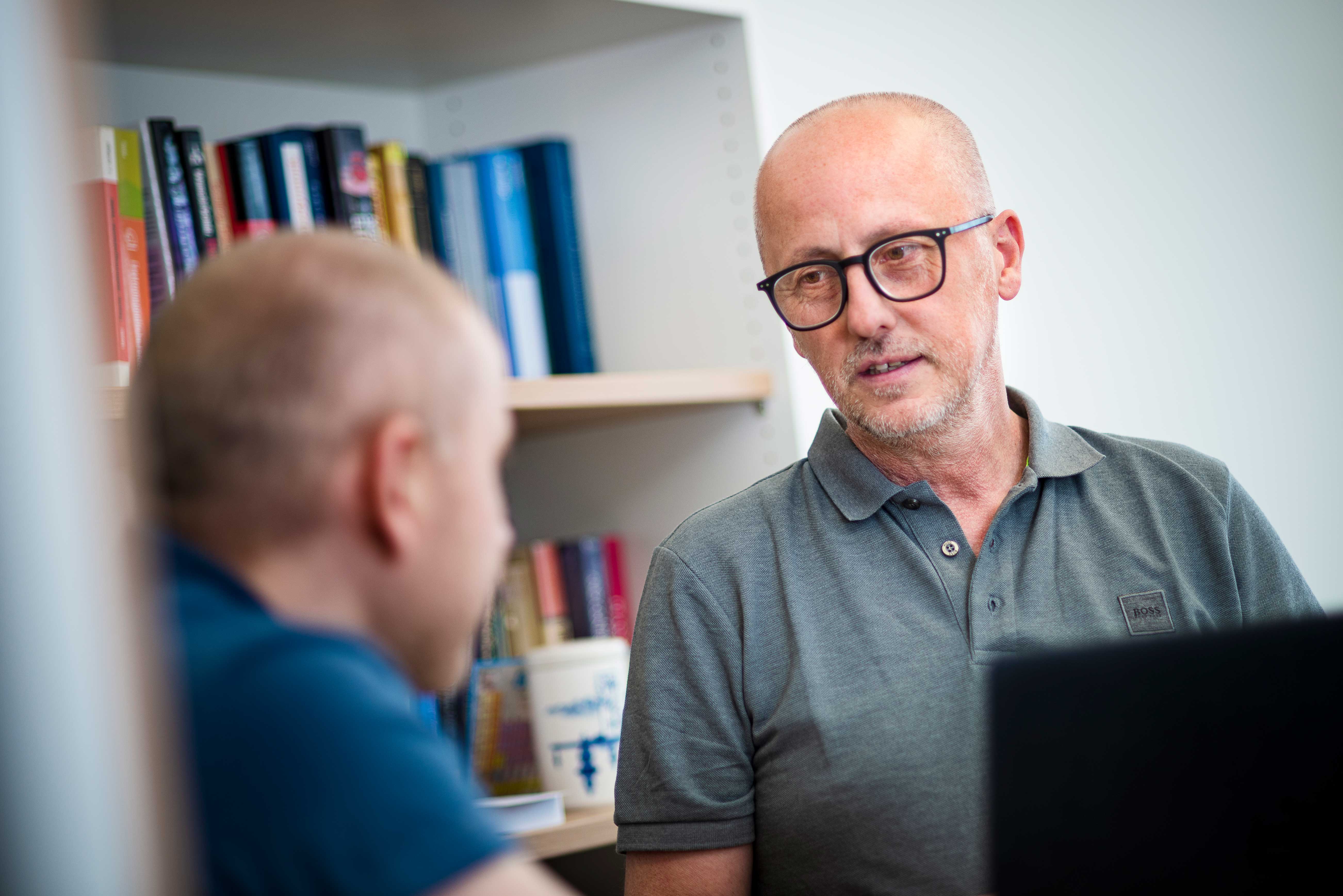
“Bringing the two ends of the time and length scales together is uncharted scientific territory,” points out Thomas Mussenbrock. Consequently, the teams are breaking new ground. Perhaps at some point, thanks to their findings, it will be possible to make large-scale industrial processes such as ammonia production more energy-efficient.
adapted from Meike Drießen (RUB)