New cosmic magnetic field structures discovered in galaxy NGC 4217
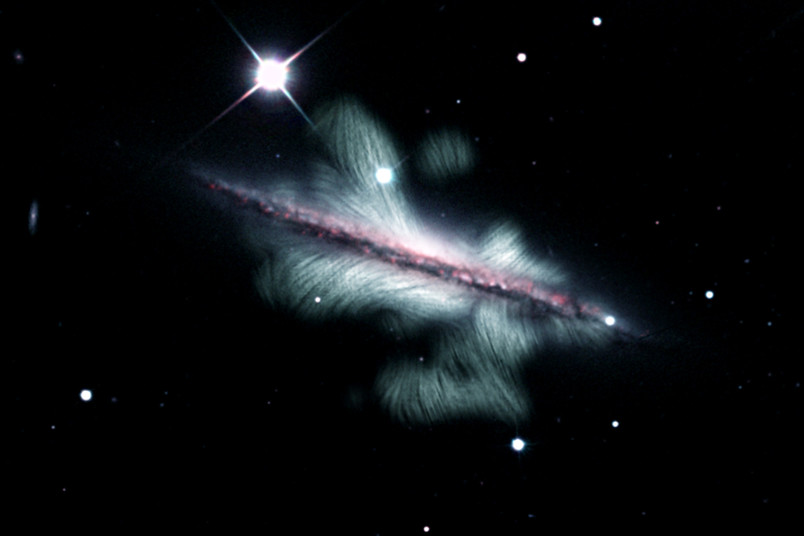
Spiral galaxies such as our Milky Way can have sprawling magnetic fields. There are various theories about their formation, but so far the process is not well understood. An international research team has now analysed the magnetic field of the Milky Way-like galaxy NGC 4217 in detail on the basis of radio astronomical observations and has discovered as yet unknown magnetic field structures. The data suggest that star formation and star explosions, so-called supernovae, are responsible for the visible structures.
The team led by Dr. Yelena Stein from Ruhr-Universität Bochum, the Centre de Données astronomiques de Strasbourg and the Max Planck Institute for Radio Astronomy in Bonn together with US-American and Canadian colleagues, published their report in the journal Astronomy and Astrophysics, released online on 21 July 2020.
The analysed data had been compiled in the project “Continuum Halos in Nearby Galaxies”, where radio waves were utilised to measure 35 galaxies. “Galaxy NGC 4217 is of particular interest to us,” explains Yelena Stein, who began the study at the Chair of Astronomy at Ruhr-Universität Bochum under Professor Ralf-Jürgen Dettmar and who currently works at the Centre de Données astronomiques de Strasbourg. NGC 4217 is similar to the Milky Way and is only about 67 million light years away, which means relatively close to it, in the Ursa Major constellation. The researchers therefore hope to successfully transfer some of their findings to our home galaxy.
Magnetic fields and origins of star formation
When evaluating the data from NGC 4217, the researchers found several remarkable structures. The galaxy has an X-shaped magnetic field structure, which has also been observed in other galaxies, extending far outwards from the galaxy disk, namely over 20,000 light years.
In addition to the X-shape, the team found a helix structure and two large bubble structures, also called superbubbles. The latter originate from places where many massive stars explode as supernovae, but also where stars are formed that emit stellar winds in the process. Researchers therefore suspect a connection between these phenomena.
“It is fascinating that we discover unexpected phenomena in every galaxy whenever we use radio polarisation measurements,” points out Dr. Rainer Beck from the MPI for Radio Astronomy in Bonn, one of the authors of the study. “Here in NGC 4217, it is huge magnetic gas bubbles and a helix magnetic field that spirals upwards into the galaxy’s halo.”
The analysis moreover revealed large loop structures in the magnetic fields along the entire galaxy. “This has never been observed before,” explains Yelena Stein. “We suspect that the structures are caused by star formation, because at these points matter is ejected outward.”
Image shows magnetic field structures
For their analysis, the researchers combined different methods that enabled them to visualise the ordered and chaotic magnetic fields of the galaxy both along the line of sight and perpendicular to it. The result was a comprehensive image of the structures.
To optimise the results, Yelena Stein combined the data evaluated by means of radio astronomy with an image of NGC 4217 that was taken in the visible light range. The image is available for download on the website public.nrao.edu. “Visualising the data was important to me,” stresses Stein. “Because when you think about galaxies, magnetic fields is not the first thing that comes to mind, although they can be gigantic and display unique structures. The image is supposed to shift the magnetic fields more into focus.”
adapted from Julia Weiler, RUB, translated by Donata Zuber
How much does the Universe weigh?
New results from physicists in Bochum have challenged the Standard Model of Cosmology.
“Since time immemorial people have been looking at the sky and trying to understand how much stars, planets, galaxies and other objects weigh,” says Professor Hendrik Hildebrandt, Heisenberg professor and head of the RUB research group Observational Cosmology. He and his team are investigating this question. More precisely, the group is not only interested in how much mass is present in the Universe, but also in its structure, i.e. whether the mass is evenly distributed in space or whether it occurs in lumps.
In order to weigh objects in the sky, cosmologists use the so-called gravitational lensing effect. When the light rays emitted by a galaxy pass massive objects on their way to Earth, they are deflected by the gravity of these objects. The heavier the object, the greater the deflection of the light beam. A galaxy whose light is deflected by the gravitational lensing effect therefore appears from Earth in a different place than it actually is. If researchers could measure the deflection, they could deduce its weight. But in order to do so, they have to overcome quite a few obstacles.
Difficulties in determining mass
“We only see the galaxy at its shifted location, but we do not know where it actually is,” as Hendrik Hildebrandt outlines one of the problems. In addition, researchers need to know the distances between the light-emitting galaxy, the deflecting mass and the observer in order to calculate the mass. “But as we only ever see a two-dimensional image of the sky, it is difficult to estimate how far away objects are along the line-of-sight,” elaborates the physicist.
Still, researchers have developed tools to address these problems. They take advantage of the fact that the massive objects do not deflect the light like perfect lenses, but create distortions. The image of a galaxy then appears as if it was viewed through the foot of a wine glass.
Gravitational lensing
Researchers can calculate these distortions; they determine the deviation from the original shape of the galaxy – naturally, they have to know its original shape in order to do so.
Average over millions of galaxies
Typically, this can’t be done for individual objects. However, researchers know what galaxies are supposed to look like on average. They therefore average over a large number of galaxies and calculate their average distortion, also known as shear. Using statistical methods, the research team determines the distortion of tens of millions of galaxies for large sections of the sky. Based on these results, the physicists can then reconstruct the deflections of light and thus the mass of the deflecting objects – provided they know the three-dimensional distances of the objects from each other.
In order to determine the density of matter in the universe using the gravitational lensing effect, cosmologists look at distant galaxies, which usually appear in the shape of an ellipse. These ellipses are randomly oriented in the sky.
In order to determine the distance of objects, the researchers use the colour of the galaxies. It has long been known that light from more distant galaxies shifts to the red as it arrives on Earth. The colour of a galaxy can thus be used to determine its distance. Cosmologists take images of galaxies at different wavelengths, for example one in the blue, one in the green, one in the red and possibly several in the infrared range. They subsequently determine the respective brightness of the galaxy in the different images. This method has long been established. “It works particularly well when you include data from the infrared range,” says Hendrik Hildebrandt, who is an expert in this type of analysis and who has introduced precisely this expertise to a project called the “Kilo-Degree Survey” – which caused quite a stir in the cosmological community.
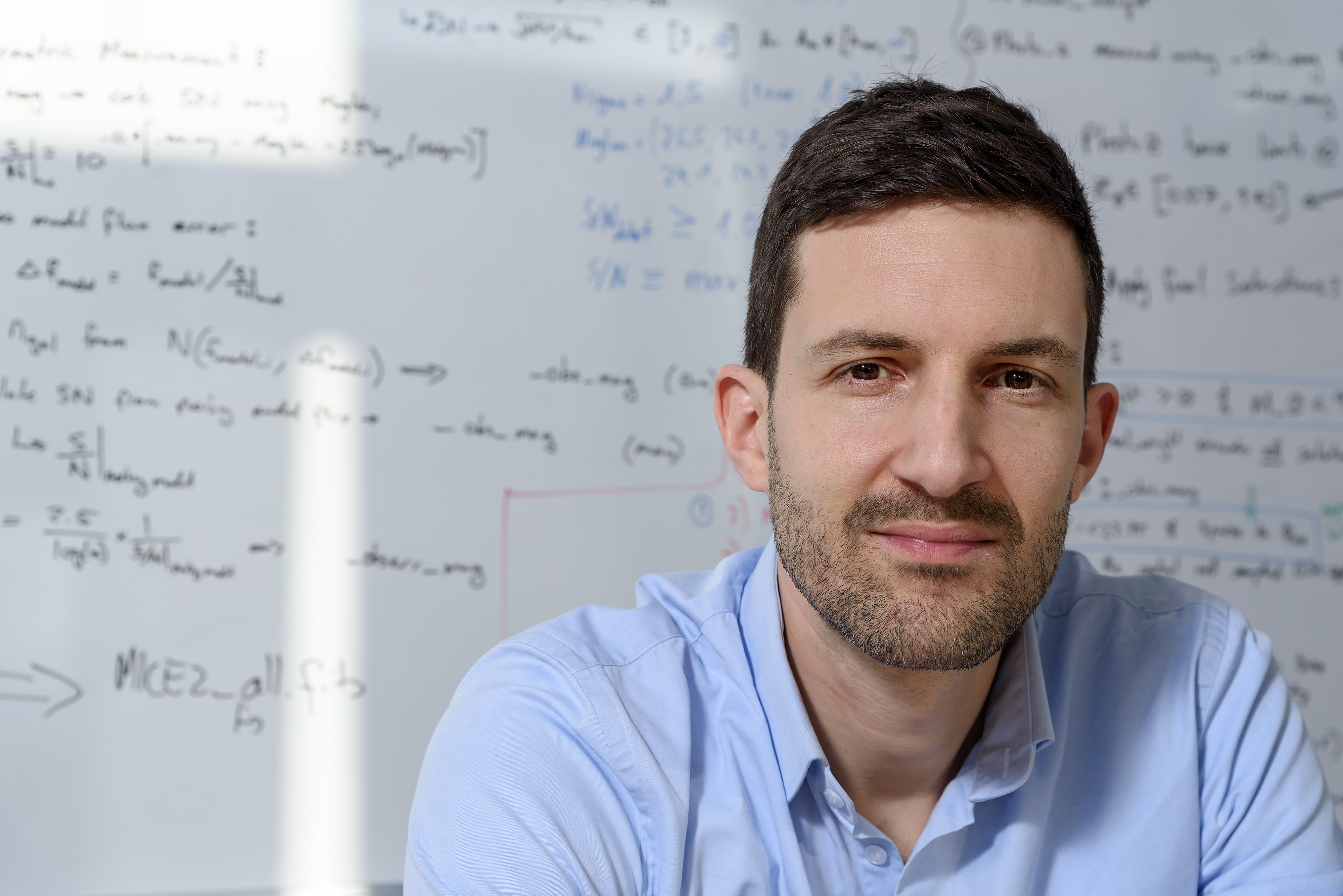
Based on the data compiled in the Kilo-Degree Survey, the research consortium determined a combined value for the density and the clumping tendency of matter in the Universe. “So far, we have been unable to distinguish clearly whether there is a lot of matter that is evenly distributed in the Universe or little matter that is extremely lumpy,” admits Hildebrandt. In the end, the analysis does not yield a single value, but rather a possible range of values into which matter density and clumping tendency might fall.
Second method for measuring the density of matter
However, scientists can measure these parameters not only with the gravitational lensing effect, as the research consortium with Hendrik Hildebrandt has done, but also with another method based on the cosmic microwave background. This refers to radiation in the microwave range, which was emitted shortly after the Big Bang and can still be measured today.
Today, values for matter density and lumpiness are available from several research consortia that used the gravitational lensing effect, as well as data from the Planck Consortium that used the cosmic microwave background. But the results do not match. Rather, the gravitational lensing measurements seem to deviate systematically from the microwave background measurements; the most obvious deviation is between the Planck Consortium and the Kilo-Degree Survey, in which Hendrik Hildebrandt is a major contributor. “There can be several reasons for this outcome,” he points out. “Either we or one of the other research consortia has made a systematic error in data evaluation – or there is something wrong with the Standard Model of Cosmology.”
This fundamental model of cosmology, based on Einstein’s general theory of relativity, describes the origin and evolution of the Universe. Researchers need it to interpret their data. “We have also included alternative models for interpretation and have actually found one that reconciles our data with those of the microwave background measurements,” says the physicist.
Standard Model of Cosmology might be wrong
In the alternative model, Einstein’s cosmological constant, which describes the gravitational force, is replaced by the so-called dark energy – a force responsible for the accelerated expansion of the Universe. “What’s interesting about the alternative model is that the dark energy in it changes over time,” explains Hendrik Hildebrandt. This could explain the discrepancy between the data sets. This is because the cosmic microwave background originates from the young Universe shortly after the Big Bang; the gravitational lensing effect, on the other hand, measures a much older Universe – the dark energy could have changed during this time span.
More extensive analysis in progress
According to Hildebrandt, it is too early yet to reject the Standard Model of Cosmology. Statistically, there is an approximately one-percent probability that the Kilo-Degree Survey data set will overlap with the Planck data. Hendrik Hildebrandt and his cooperation partners therefore intend to determine the density and lumpiness of matter even more precisely than before and are currently evaluating a more comprehensive data set. “It remains to be seen whether, after this analysis, our data will be even less compatible with the Planck Consortium’s data or whether they can both be reconciled,” he says.
Either way, this is a pivotal moment for the Bochum-based researcher. “It is the first time in my research career that I have reached such a critical point,” he stresses. “The noblest task of an experimental physicist is to bring down theories.” Now the Bochum-based team is eagerly waiting to see whether the explanation for the discrepancy in the data will be a quite mundane one, namely a measurement error. “But it is quite possible that we will trigger a revolution with our new data,” concludes Hildebrandt. The team expects the results to be released in late spring 2020.
The Very Large Telescope (in the background) is located on Paranal in northern Chile in the Atacama Desert, which offers optimal conditions for astronomical observations. In the foreground is the Vista telescope that supplies the infrared data.
written by Julia Weiler, RUB
Sciencific magazine of RUB asks for definition of a body
The scientific magazine of the Ruhr-University Bochum had a series of interview concerning the definition of a body within its last edition. Two members of the Reserach Department Plasmas with Complex Interactions gave their opinion about this topic.
Astronomy
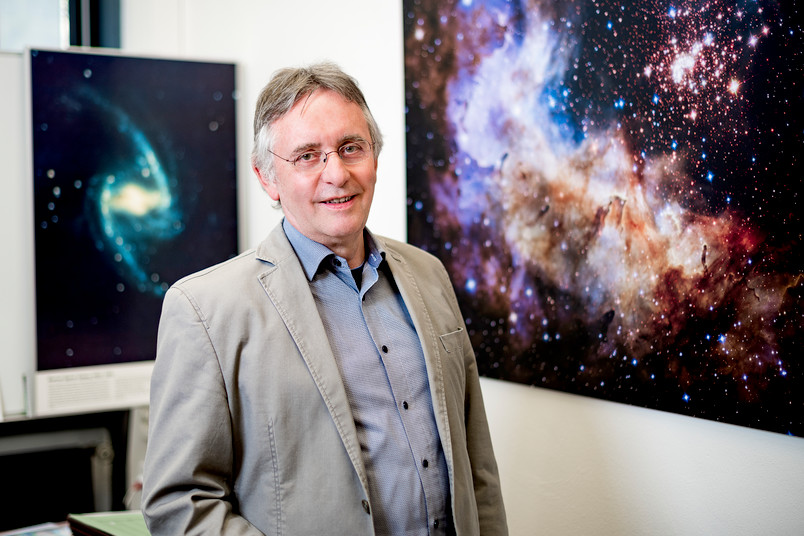
As an astronomer, I associate the term "body" most closely with celestial bodies. This is generally associated with objects that Kant defined in 1755 as "... round masses, thus of the simplest formation that a body whose origin is sought can only always have". At that time these were - in extension of "sun, moon and stars" - mainly the classical planets. But actually, I am fascinated by other celestial bodies at the moment, which do not always correspond to the idea of a "round mass" and of which smaller specimens can even be picked up: meteorites. The shape can look like a wrinkled, dried-up potato. The smooth, uniform outer layer hardly shows the important inside. One may wonder how the objects of our planetary system could have developed from these four billion year old lumps. The RUB Mineralogical Collection contains some interesting examples of these small celestial bodies.
Prof. Dr. Ralf-Jürgen Dettmar
Physics
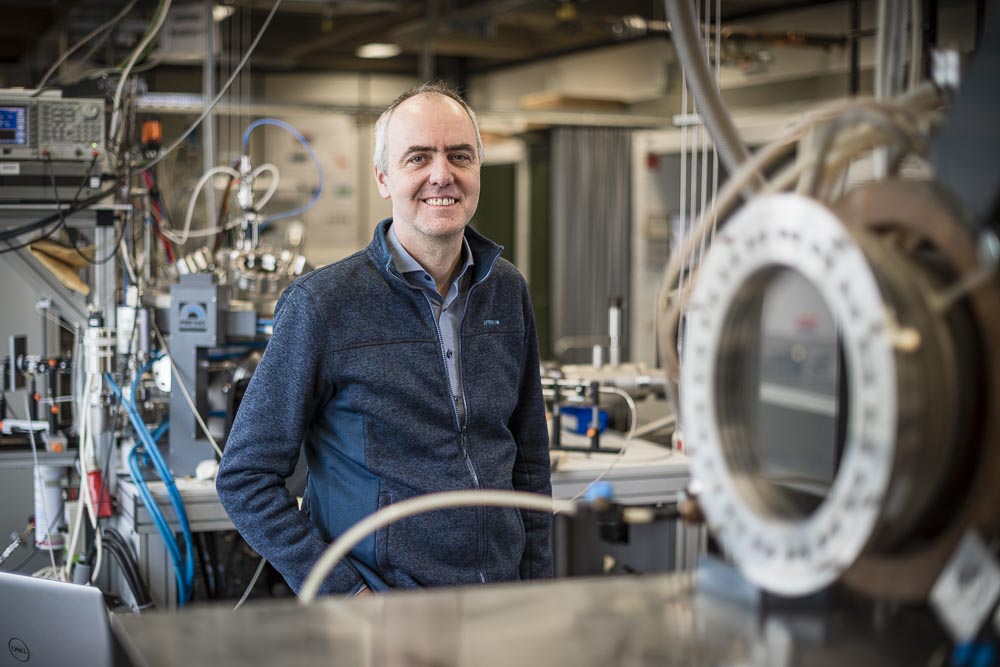
What is a body? The question is wrongly posed from the perspective of physics. In everyday life we take the bodies in our environment through our senses. There is an interaction of particles with this body, which provides us with information about its spatial extension: be it the light particles when we see or the atoms on the surface of our hand when we touch it. The kind of interaction determines the extension of a body, which is investigated in atomic and nuclear physics in scattering expe- rents. This is done by bombarding atoms or atomic nuclei with certain particles and measuring their deflection and energy loss. Electrons, protons, neutrons or light particles are used as projectiles - and depending on the type of projectile and its energy, the results vary considerably. With neutrons, only the atomic nucleus is visible, with electrons and light particles rather the atomic shell. Thus the question of the size of an atom or atomic nucleus has many answers. This interaction is also important on a large scale. For example, the size of a black hole is defined more by the limit of the interaction of light with gravity than by the spatial extension of the heavy celestial body in its interior.
These types of interaction determine how we deal with bodies in everyday life. Thus, holding this ruby booklet with your hands is, in a sense, the interaction of the atoms of your hand with the cellulose of the paper from which this booklet is made. One of the most important laws of quantum physics, the Pauli prohibition, determines that the atoms of your hands cannot easily penetrate those of the paper. Therefore, the notebook lies stable in your hands and you can perceive this body in its size.
Prof. Dr. Achim von Keudell
Plasma-driven biocatalysis
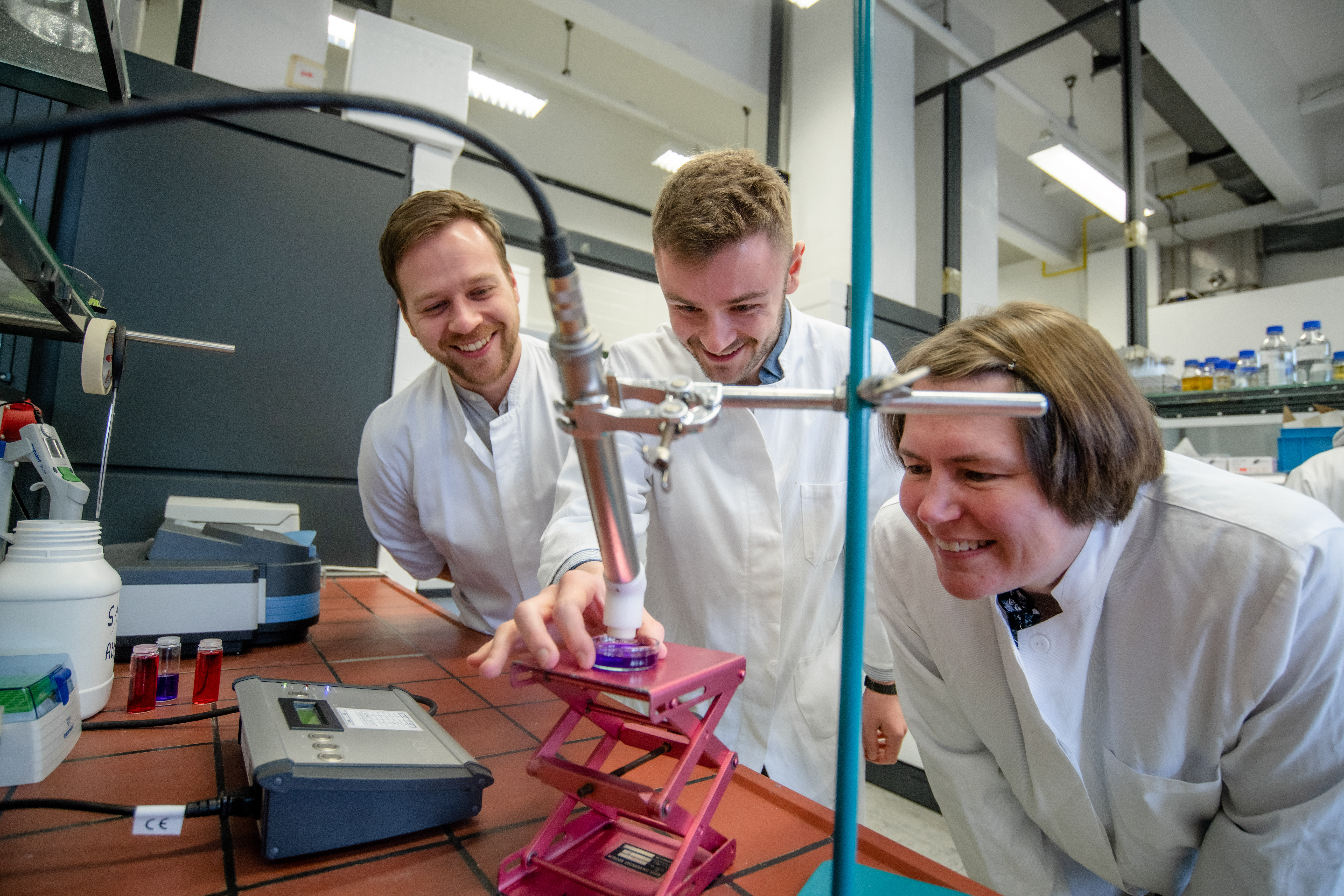
A research team from Bochum has developed a new method to drive catalytically active enzymes.
Compared with traditional chemical methods, enzyme catalysis has numerous advantages. But it also has weaknesses. Some enzymes are not very stable. Enzymes that convert hydrogen peroxide are even inactivated by high concentrations of the substrate. A research team at Ruhr-Universität Bochum (RUB), together with international partners, has developed a process in which the starting material, i.e. hydrogen peroxide, is fed to the biocatalysts in a controlled manner using plasma. The enzymes themselves are protected from harmful components of the plasma by a buffer layer. Using two model enzymes, the team showed that the process works, as reported in the journal “ChemSusChem” from 5 February 2020.
Milder conditions, less energy consumption and waste
In biocatalysis, chemicals are produced by cells or their components, in particular by enzymes. Biocatalysis has many advantages over traditional chemical processes: the reaction conditions are usually much milder, energy consumption is lower and less toxic waste is produced. The high specificity of enzymes also means that fewer side reactions occur. Moreover, some fine chemicals can only be synthesised by biocatalysis.
The weak spot of enzyme biocatalysis is the low stability of some enzymes. “Since the enzyme often has to be replaced in such cases – which is expensive – it is extremely important to increase the stability under production conditions,” explains lead author Abdulkadir Yayci from the Chair of Applied Microbiology headed by Professor Julia Bandow.
Hydrogen peroxide: necessary, but harmful
The research team has been studying two similar classes of enzymes: peroxidases and peroxygenases. Both use hydrogen peroxide as a starting material for oxidations. The crucial problem is that hydrogen peroxide is absolutely necessary for activity, but in higher concentrations it leads to a loss of activity of the enzymes. As far as these enzyme classes are concerned, it is therefore vital to supply hydrogen peroxide in precise doses.
To this end, the researchers investigated plasmas as a source of hydrogen peroxide. Plasma describes the fourth state of matter that is created when energy is added to a gas. If liquids are treated with plasmas, a large number of reactive oxygen and nitrogen species are formed, some of which then react to form long-lived hydrogen peroxide, which can be used for biocatalysis.
Biocatalytic reactions with plasma-generated hydrogen peroxide are possible
In an experiment in which horseradish peroxidase served as one of the model enzymes, the team showed that this system works in principle. At the same time, the researchers identified the weak points of plasma treatment: “Plasma treatment also directly attacks and inactivates the enzymes, most likely through the highly reactive, short-lived species in the plasma-treated liquid,” outlines Abdulkadir Yayci. The research group improved the reaction conditions by binding the enzyme to an inert carrier material. This creates a buffer zone above the enzyme in which the highly reactive plasma species can react without harming the enzyme.
The researchers then tested their approach using a second enzyme, the unspecific peroxygenase from the fungus Agrocybe aegerita. This peroxygenase has the ability to oxidise a large number of substrates in a highly selective way. “We successfully demonstrated that this specificity is maintained even under plasma treatment and that highly selective biocatalytic reactions are possible using plasma,” concludes Julia Bandow.
written by Marike Drießen, RUB
Lightning bolt underwater
A plasma tears through the water within a few nanoseconds. It may possibly regenerate catalytic surfaces at the push of a button.
Electrochemical cells help recycle CO2. However, the catalytic surfaces get worn down in the process. Researchers at the Collaborative Research Centre 1316 “Transient atmospheric plasmas: from plasmas to liquids to solids” at Ruhr-Universität Bochum (RUB) are exploring how they might be regenerated at the push of a button using extreme plasmas in water. In a first, they deployed optical spectroscopy and modelling to analyse such underwater plasmas in detail, which exist only for a few nanoseconds, and to theoretically describe the conditions during plasma ignition. They published their report in the journal Plasma Sources Science and Technology on 4 June 2019. A plasma tears through the water within a few nanoseconds. Following plasma ignition, there is a high negative pressure difference at the tip of the electrode, which results in ruptures forming in the liquid. Plasma then spreads across those ruptures.
Plasmas are ionised gases: they are formed when a gas is energised that then contains free electrons. In nature, plasmas occur inside stars or take the shape of polar lights on Earth. In engineering, plasmas are utilised for example to generate light in fluorescent lamps, or to manufacture new materials in the field of microelectronics. “Typically, plasmas are generated in the gas phase, for example in the air or in noble gases,” explains Katharina Grosse from the Institute for Experimental Physics II at RUB.
Ruptures in the water
In the current study, the researchers have generated plasmas directly in a liquid. To this end, they applied a high voltage to a submerged hairline electrode for the range of several billionth seconds. Following plasma ignition, there is a high negative pressure difference at the tip of the electrode, which results in ruptures forming in the liquid. Plasma then spreads across those ruptures. “Plasma can be compared with a lightning bolt – only in this case it happens underwater,” says Katharina Grosse.
Hotter than the sun
Using fast optical spectroscopy in combination with a fluid dynamics model, the research team identified the variations of power, pressure, and temperature in these plasmas. “In the process, we observed that the consumption inside these plasmas briefly amounts to up to 100 kilowatt. This corresponds with the connected load of several single-family homes,” points out Professor Achim von Keudell from the Institute for Experimental Physics II. In addition, pressures exceeding several thousand bars are generated – corresponding with or even exceeding the pressure at the deepest part of the Pacific Ocean. Finally, there are short bursts of temperatures of several thousand degrees, which roughly equal and even surpass the surface temperature of the sun.
Water is broken down into its components
Such extreme conditions last only for a very short time. “Studies to date had primarily focused on underwater plasmas in the microsecond range,” explains Katharina Grosse. “In that space of time, water molecules have the chance to compensate for the pressure of the plasma.” The extreme plasmas that have been the subject of the current study feature much faster processes. The water can’t compensate for the pressure and the molecules are broken down into their components. “The oxygen that is thus released plays a vital role for catalytic surfaces in electrochemical cells,” explains Katharina Grosse. “By re-oxidating such surfaces, it helps them regenerate and take up their full catalytic activity again. Moreover, reagents dissolved in water can also be activated, thus facilitating catalysis processes.”
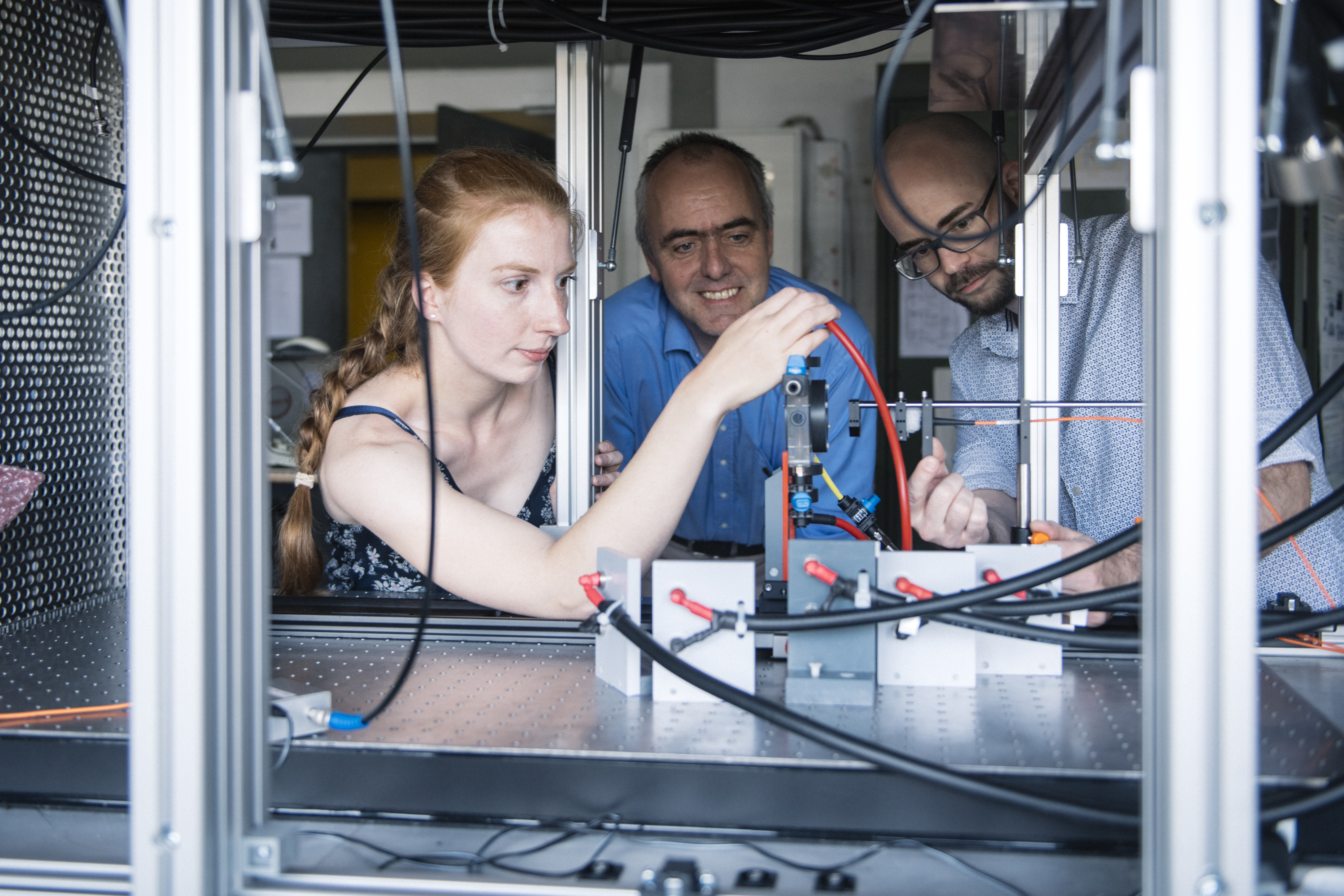
By Meike Drießen, Translated by Donata Zuber
How bacteria protect themselves from plasma treatment
Plasmas are applied in the treatment of wounds to combat pathogens that are resistant against antibiotics. But bacteria know how to defend themselves.
Considering the ever-growing percentage of bacteria that are resistant to antibiotics, interest in medical use of plasma is increasing. In collaboration with colleagues from Kiel, researchers at Ruhr-Universität Bochum (RUB) investigated if bacteria may become impervious to plasmas, too. They identified 87 genes of the bacterium Escherichia coli, which potentially protect against effective components of plasma. “These genes provide insights into the antibacterial mechanisms of plasmas,” says Marco Krewing. He is the lead author of two articles that were published in the Journal of the Royal Society Interface this year.
A cocktail of harmful components stresses pathogens
Plasmas are created from gas that is pumped with energy. Today, plasmas are already used against multi-resistant pathogens in clinical applications, for example to treat chronic wounds. “Plasmas provide a complex cocktail of components, many of which act as disinfectants in their own right,” explains Professor Julia Bandow, Head of the RUB research group Applied Microbiology. UV radiation, electric fields, atomic oxygen, superoxide, nitric oxides, ozone, and excited oxygen or nitrogen affect the pathogens simultaneously, generating considerable stress. Typically, the pathogens survive merely several seconds or minutes.
In order to find out if bacteria, may develop resistance against the effects of plasmas, like they do against antibiotics, the researchers analysed the entire genome of the model bacterium Escherichia coli, short E. coli, to identify existing protective mechanisms. “Resistance means that a genetic change causes organisms to be better adapted to certain environmental conditions. Such a trait can be passed on from one generation to the next,” explains Julia Bandow.
Mutants missing single genes
For their study, the researchers made use of so-called knockout strains of E. coli. These are bacteria that are missing one specific gene in their genome, which contains approximately 4,000 genes. The researchers exposed each mutant to the plasma and monitored if the cells kept proliferating following the exposure.
“We demonstrated that 87 of the knockout strains were more sensitive to plasma treatment than the wild type that has a complete genome,” says Marco Krewing. Subsequently, the researchers analysed the genes missing in these 87 strains and determined that most of those genes protected bacteria against the effects of hydrogen peroxide, superoxide, and/or nitric oxide. “This means that these plasma components are particularly effective against bacteria,” elaborates Julia Bandow. However, it also means that genetic changes that result in an increase in the number or activity of the respective gene products are more capable of protecting bacteria from the effects of plasma treatment.
Heat shock protein boosts plasma resistance
The research team, in collaboration with a group headed by Professor Ursula Jakob from the University of Michigan in Ann Arbor (USA), demonstrated that this is indeed the case: the heat shock protein Hsp33, encoded by the hslO gene, protects E. coli proteins from aggregation when exposed to oxidative stress. “During plasma treatment, this protein is activated and protects the other E. coliproteins – and consequently the bacterial cell,” Bandow points out. An increased volume of this protein alone results in a slightly increased plasma resistance. Considerably stronger plasma resistance can be expected when the levels of several protective proteins are increased simultaneously.
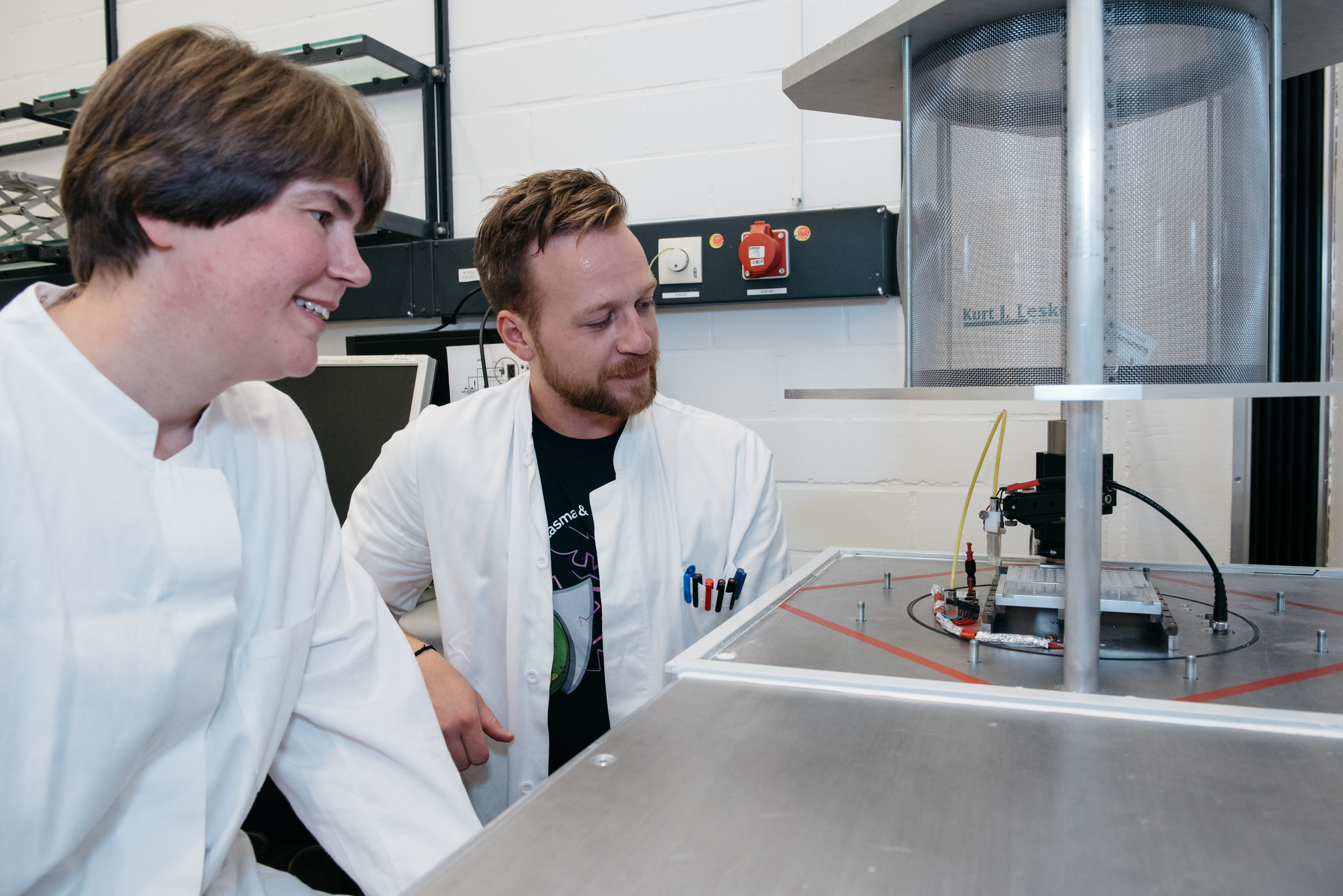
By Meike Drießen, Translated by Donata Zuber
New class of catalysts for energy conversion
The research group of Prof. Ludwig and his colleagues recently published their new results in the catalyst production. “At our department, we have unique methods at our disposal to manufacture these complex materials from five source elements in different compositions in form of thin film or nanoparticle libraries,” explains Professor Alfred Ludwig from the Chair of Materials for Microtechnology at RUB. The atoms of the source elements blend in plasma and form nanoparticles in a substrate of ionic liquid. If the nanoparticles are located in the vicinity of the respective atom source, the percentage of atoms from that source is higher in the respective particle. “Very limited research has as yet been conducted into the usage of such materials in electrocatalysis,” says Ludwig.
The full press releas can be found here.
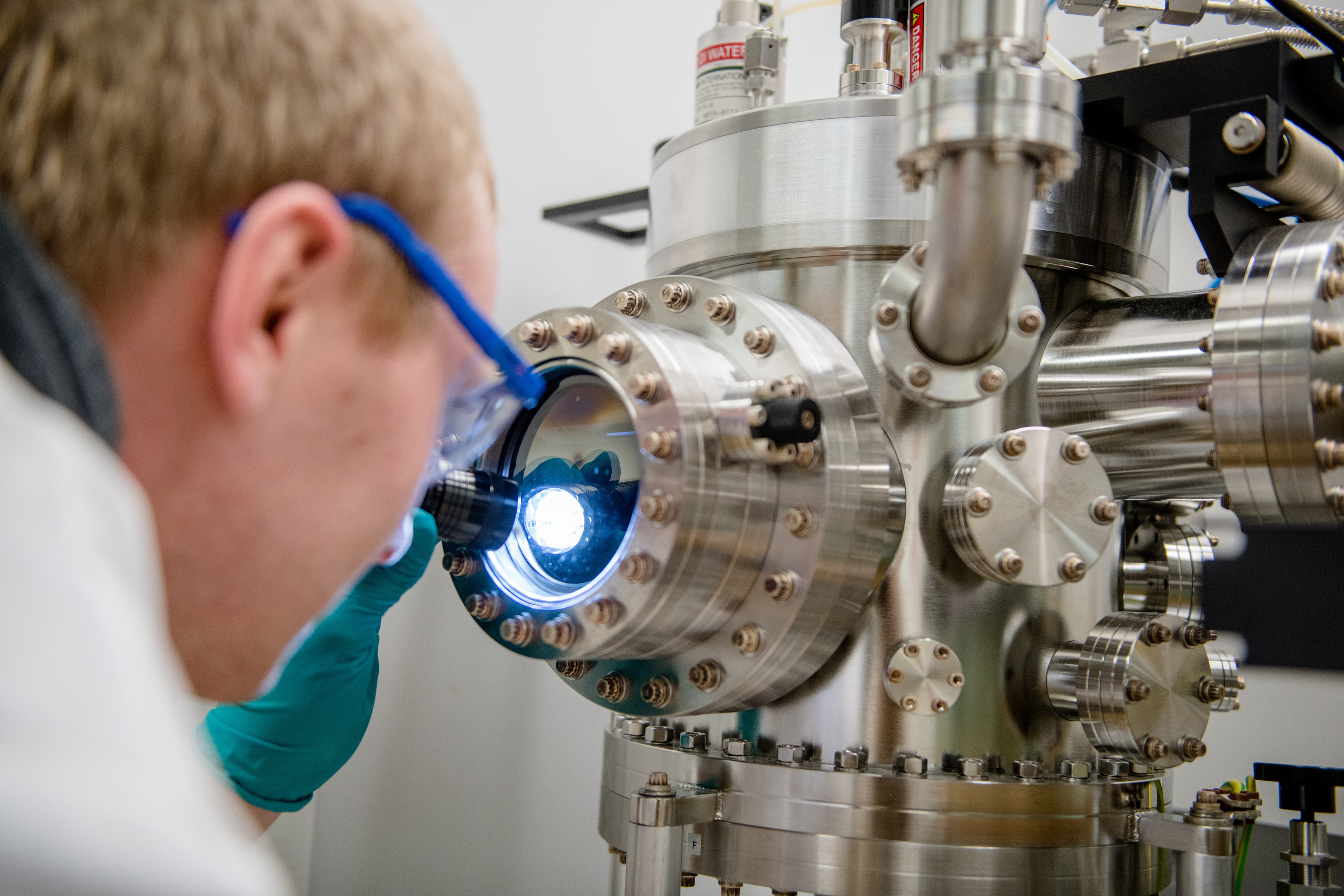
A new map of the sky with hundreds of thousands of galaxies
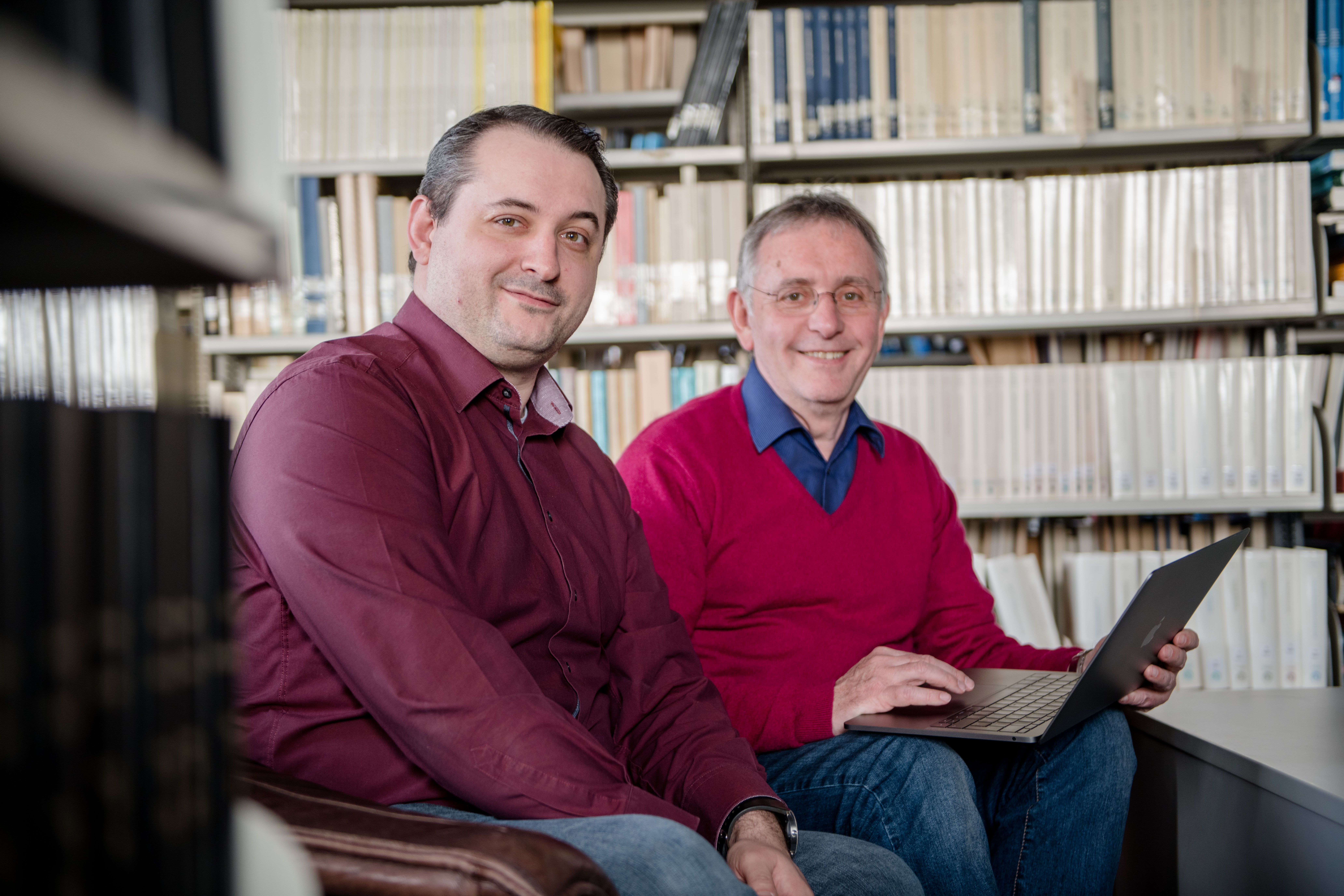
The group of Prof. Dettmar from the Astronomical Insitute contribute to the special issue of the journal "Astronomy & Astrophysics".
A team of astronomers from the Ruhr-Universität Bochum (RUB) has studied one of the discovered galaxies in detail and found a characteristic radiation distribution that suggests processes in the formation of galaxies and our Milky Way.
The Lofar Telescope
Lofar is a vast European network of radio telescopes linked together by a high-speed fibre-optic network, whose measurement signals are combined into a single signal. Powerful supercomputers convert 100,000 individual antennas into a virtual reception dish with a diameter of 1,900 kilometers. Lofar operates in the frequency ranges between about 10 to 80 megahertz and 110 to 240 megahertz, which have so far been largely unexplored. It is controlled by the Astron research facility in the Netherlands and is considered the world's leading telescope of its kind. There are six measuring stations in Germany which are operated by various scientific institutions.
Supernovae influence the evolution of galaxies
Dr. Arpad Miskolczi of the RUB Chair of Astronomy is one of the first authors of the collection of research results, all based on the analysis of a first phase of the multi-year project. In collaboration with international colleagues, he has investigated one of the many newly discovered galaxies in more detail. The object with the catalogue name NGC 3556 shows a characteristically different radiation distribution in the radio range than in visible light. "From this we conclude that the accumulation of numerous huge stellar explosions, so-called supernovae, releases so much energy that the gas between the stars, interspersed with magnetic fields and particles of cosmic rays, leaves the galaxy,"; explains Prof. Dr. Ralf-Jürgen Dettmar. These processes have influenced the evolution of Milky Way systems over billions of years. By comparing different such objects, the researchers hope to gain information about the origin of our own Milky Way.
Black holes, magnetic fields, galaxy clusters
With the help of Lofar, scientists have been able to create a new sky map. Many of the galaxies depicted were previously unknown because they are extremely far away and their radio signals have to travel billions of light years to reach Earth.
When scientists observe the sky with a radio telescope, they mainly see radiation from the vicinity of black holes, which are millions of times heavier than the sun. With Lofar, the researchers want to find out what influence the black holes have on the galaxies in which they are located and where they come from. Thanks to Lofar's sensitivity, the teams have already been able to show that black holes are present in all giant galaxies and that they are constantly growing.
The radio radiation received by Lofar can also be used to measure cosmic magnetic fields. The researchers have also been able to detect magnetic structures between galaxies, thus proving theoretical assumptions for the first time.
The fusion of two clusters of galaxies produces radio emissions - so-called radio halos - with a size of millions of light years. With Lofar they can be tracked down. The researchers learn a lot from this about the gas at the edge of the gigantic clusters of galaxies.
Gigantic amounts of data
The creation of low frequency radio sky maps requires both considerable telescope and computational time and requires analysis of the data by large teams. "Lofar produces gigantic amounts of data - we have to process the equivalent of ten million DVDs. This places the highest demands on software and hardware and is only possible through an international and interdisciplinary team,"; says Prof. Dr. Dominik Schwarz of Bielefeld University and representative of Germany to the Lofar steering committee.
"In Germany, we worked together with Forschungszentrum Jülich to efficiently convert the huge amounts of data into high-quality images. These images are now public and will allow astronomers to study the evolution of galaxies in unprecedented detail,"; adds Prof. Dr. Ralf-Jürgen Dettmar.
The Forschungszentrum Jülich accommodates around 15 petabytes of Lofar data. "This is almost half of all Lofar data, one of the largest astronomical data collections in the world. The processing of these gigantic data sets represents a great challenge. What would have taken centuries on conventional computers could have been reduced to one year by using innovative algorithms and extremely powerful computers,"; says Prof. Dr. Dr. Thomas Lippert, Director of the Jülich Supercomputing Centre. Jülich is one of the three data centres of the Lofar project. In addition, the Jülich Supercomputing Centre manages the data network traffic between the German Lofar stations and the central Lofar computer in Groningen.
15 million radio sources expected
The 26 papers now published in a special issue of Astronomy & Astrophysics are based on only about two percent of the observations planned with Lofar. The scientists now want to map the entire northern celestial sphere. After all, they expect to find around 15 million radio sources.
The original press releases can be found here.
Ultra-thin transparent silver layers for solar cells
New chemical precursor
"The precursors for the deposition of ultrathin silver layers are extremely sensitive to air and light," explains Nils Boysen. It is true that the precursors can be stabilized with fluorine, phosphorus or oxygen. "However, these elements contaminate the thin film and pollute the equipment used to produce the layers," the researcher continues. As part of his master's thesis, Boysen and colleagues developed an alternative for stabilization.
The scientists designed a chemical silver precursor in which the silver is packaged in an amide and a carbene, thus eliminating the need for stabilization with fluorine, phosphorus or oxygen. They showed that the new precursor can be used to deposit thin silver layers on an electrode. To do this, they used atomic layer deposition. In the process, the precursor is transported to the electrode in gaseous form and deposited there as a silver layer just a few atoms thick. Because the silver film is so thin, it is transparent.
"Since the process works at atmospheric pressure and low temperatures, the general conditions would be favorable for industrial production," says Anjana Devi.
Prospect of high-efficiency solar cells and lights
With a series of tests, the researchers demonstrated that the silver thin films produced with the new process are pure and electrically conductive. "From a process engineering point of view, the successful synthesis of the new precursor opens the door to realize ultrathin silver films," Thomas Riedl sums up. "They hold out the prospect of producing new electrodes for highly efficient solar cells and lights."
"The interdisciplinary collaboration between the Bochum materials scientists and the Wuppertal engineers was the key to success," says Anjana Devi.
adapted from Julia Weiler (RUB)
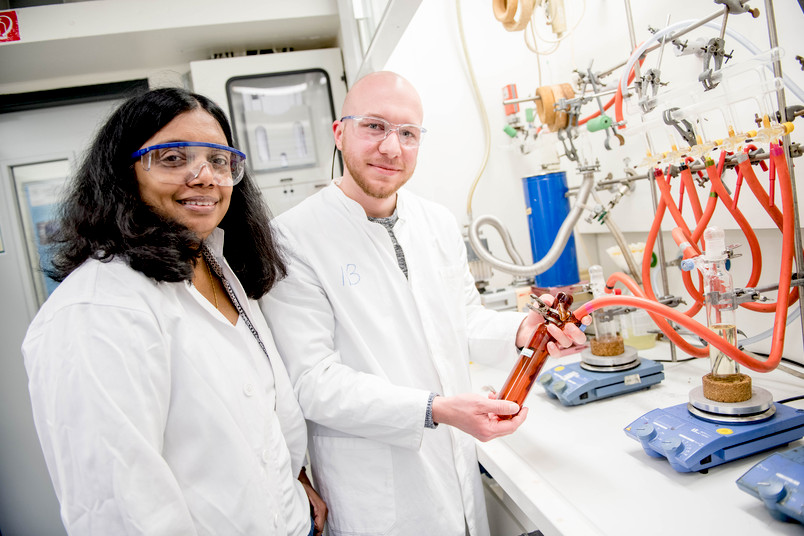
Three Collaborative Research Centers enter the third funding phase
Collaborative Research Center/Transregio 87 "Pulsed high-power plasmas for the synthesis of nanostructured functional layers".
The path to new materials on the surface of classic materials, for example for electromobility, energy technology - for solar cells, for example - but also medical technology, for example for packaging and sterilization, leads via plasma technology. Researchers from plasma technology, plasma physics, materials science and interfacial chemistry work together in the Collaborative Research Center/Transregio 87 "Pulsed High-Power Plasmas for the Synthesis of Nanostructured Functional Layers".
The subject matter includes, for example, ceramic layer systems on metal substrates and silicon or carbon-containing oxide layers on plastic substrates. "For this purpose, we use the latest source technology, some of which we have developed ourselves, and investigate the resulting layers with a broad spectrum of plasma diagnostics, some of which have also been newly developed, and unique single-particle beam experiments," explains spokesman Prof. Dr. Peter Awakowicz.
The research focuses on pulsed high-power plasmas. The aim is to explore and quantify the relationships between material properties and plasma parameters and to use them for plasma control, layer development and coating control. In this way, the researchers aim to overcome the empirical approach that has predominated to date and develop a physically and chemically based understanding of the process.
In the coming funding phase, the participants intend to make the knowledge and methods gained so far available to other coating processes, reactor systems, surface and material systems, and computer codes.
adapted from Meike Drießen (RUB)
Astonishing order in space
A fine wire, often barely discernible, is the only part of the radio telescopes used for observing the sky in Jülich that is visible above ground. There are no impressive bowls – just metal threads arranged in the shape of pyramids and located in a meadow. “These are perfectly ordinary wires, such as are also used as antennas in radios,” explains Prof Dr Ralf-Jürgen Dettmar, whose team was involved in the construction of the Lofar radio telescope network, short for Low Frequency Array, at the research centre Forschungszentrum Jülich. Astronomers utilise them to capture radio emissions from remote galaxies. Jülich is one of many European locations that feed data into a shared network, the centre of which is situated in the Dutch town of Groningen.
Digital technology
Even though the telescope antennas may seem unremarkable at first glance, they are part of a high-tech system. “Today, all data are digitalised, which is why combining and correlating signals supplied by several antennas is easily done,” explains Ralf-Jürgen Dettmar, who heads the RUB Chair of Astronomy. “We have thus pushed the envelope in terms of the things that we can observe.”
This is because modern technology enables researchers to record data at greater sensitivity and from a broader frequency band than it had been possible in the pre-digital era, when small cables were all that was available to establish connections within a network. Moreover, this approach yields a greater data output than hitherto. “We now have 1,024 or 2,048 reading points or more in instances where we used to have only one,” elaborates Dettmar.
All new radio telescopes are based on digital technology. The RUB group is involved in various large-scale research projects worldwide, developing novel methods for data analysis and researching into specific cosmic phenomena, such as galactic magnetic fields that can be analysed indirectly with the aid of radio telescopes.
Galactic magnetic fields
The radio emissions are caused by the following mechanism: free electrons are accelerated in giant stellar explosions and, because of their charge, perform rapid precession in the cosmic magnetic field. In the process, they emit radiation at radio wave frequencies. As the precession is linked to the magnetic field, the emissions contain information on the magnetic field itself. Point by point, the telescopes record an image of spectral radiance in a specific cosmic region, enabling the researchers to calculate the strength and direction of the magnetic field based on the radio signals.
It had previously been assumed that the magnetic fields are trapped in the disc.
– Ralf-Jürgen Dettmar
Using this method, Ralf-Jürgen Dettmar’s team has compiled new information on galactic magnetic fields. “Galaxies such as our Milky Way are relatively flat objects, which we should picture as discs,” he describes. “It had previously been assumed that the magnetic fields are trapped in the disc.” However, the astronomers from Bochum have proved that this is not the case.
Large-scale structures emerge from chaotic processes
“We have demonstrated that the magnetic field protrudes from the disc,” points out Dettmar. In the same way as the terrestrial magnetic field surrounds our planet, the galactic magnetic field stretches from the disc all the way to the intergalactic medium, i.e. into the space between galaxies. “The truly surprising aspect was: the magnetic fields of the first galaxy we’d studied present a regular structure on the large scale,” says the astronomer.
Considering the origins of magnetic fields, this comes as a surprise. They are generated through the turbulent process of stellar explosions. Stars in a galaxy perish in supernovas again and again, and the effects of such explosions last for millions of years. The energy bursts of all supernovas put together produce a galaxy’s magnetic fields. The researches were astonished that a process as chaotic as that results in a magnetic field that is orderly on a large scale. The underlying mechanisms have not yet been fully understood.
By no means an isolated case
In the first step, the Bochum-based astronomers had to demonstrate that what they’d observed was not an isolated case. “Seeing as measuring techniques have been considerably optimised in the wake of digitalisation, we were able to perform larger spot checks,” says Ralf-Jürgen Dettmar. On their first attempt, the researchers were unable to analyse more than three galaxies. Today, they have data of 40 galaxies at their disposal, which have been recorded by a telescope in North America over a period of 400 hours. The records were gathered in 2011 and 2012; now, more than five years later, the analyses have yielded first results.
“Following the digitalisation process, so much data has been accumulated that we required appropriate equipment to be able to handle it at all,” relates Dettmar. Old analysis methods were no longer sufficient; new algorithms became necessary, in order to process the large data volumes and calculate the intensity and direction of magnetic fields.
Causes of large-scale structure unclear
Researchers draw conclusions regarding the intensity of magnetic fields based on the intensity of the measured radio emissions. They calculate the direction of magnetic fields based on the polarisation direction of the incoming radiation. “The radiation is emitted from a turbulent medium, where particles swirl at high velocity,” describes the astronomer from Bochum. “The magnetic field lines, too, are interwoven, and it is not easy to calculate directions.” Nevertheless, large-scale structures emerged very clearly in the galactic magnetic fields.
Almost all analysed galaxies show a large-scale magnetic field; there are, however, a few outliers where no such structures can be discerned. Furthermore, the structure is not identical in all galaxies. “For now we can only speculate about the reasons of this phenomenon,” says Dettmar. “It might have something to do with the type of the respective galaxy. But we don’t know that yet.” He and his research team will continue to investigate this question, as well as several other mysteries associated with cosmic magnetic fields.
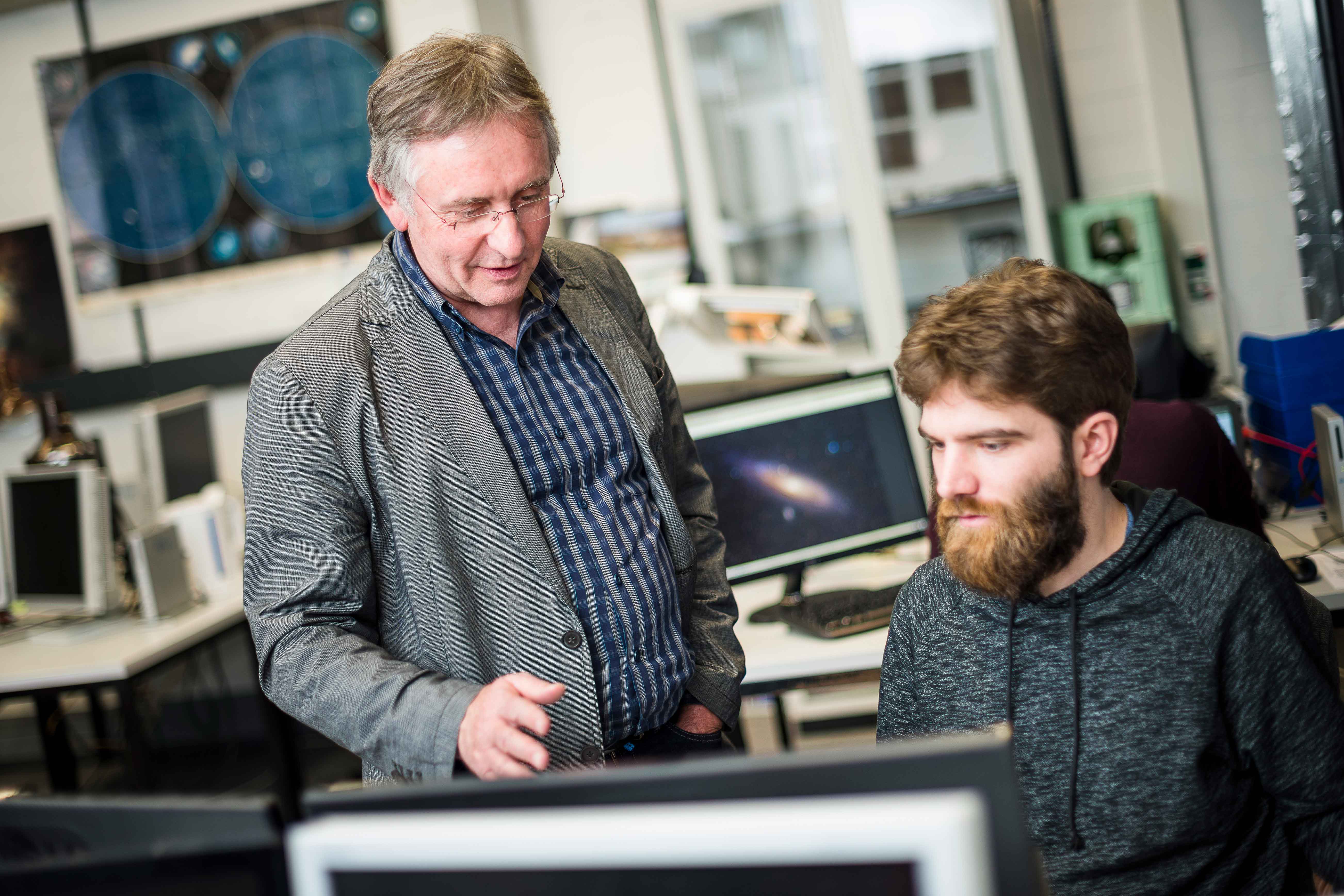
A question of sensitivity
The size of the magnetic field surrounding a galaxy, for example, is still unclear. “We haven’t yet spotted an edge,” explains Dettmar. The greater the distance to the galaxy, the weaker the magnetic field – consequently, observing it becomes increasingly difficult. The longer the astronomers point their telescopes at the magnetic field, the more sensitive the measurement becomes, because the signals are integrated over time. This means: when researchers observe a galaxy over a long period of time, they see larger magnetic fields, because they are able to detect the weaker regions, too. So far, they have not identified an end.
This is about the limit of what we can do.
– Ralf-Jürgen Dettmar
Dettmar hopes that the technology will one day be sensitive enough to figure out the nature of intergalactic magnetic fields. “According to some theories, the intergalactic medium is suffused with magnetic fields, but they must be considerably weaker than the galactic fields,” he says. “We cannot observe them directly as yet; this is about the limit of what we can do.”
Intersection with other disciplines
A detailed understanding of magnetic fields in space might help fathom other cosmic phenomena, including the question how matter spreads across galaxies the way we observe today, or what are the sources of cosmic radiation.
Such questions at the intersection of several disciplines are investigated by researchers at the Ruhr Astroparticle Plasma Physics Center, of which Ralf-Jürgen Dettmar is also a member. The institution is home to 100 scientists from all across the Ruhr area who research into astrophysics, particle physics, and plasma physics. With joined forces, they intend to find answers to questions that one discipline alone is unable to solve.
adapted from Julia Weiler (RUB)
A man for the very special prices
adapted from Arne Dessaul (RUB)
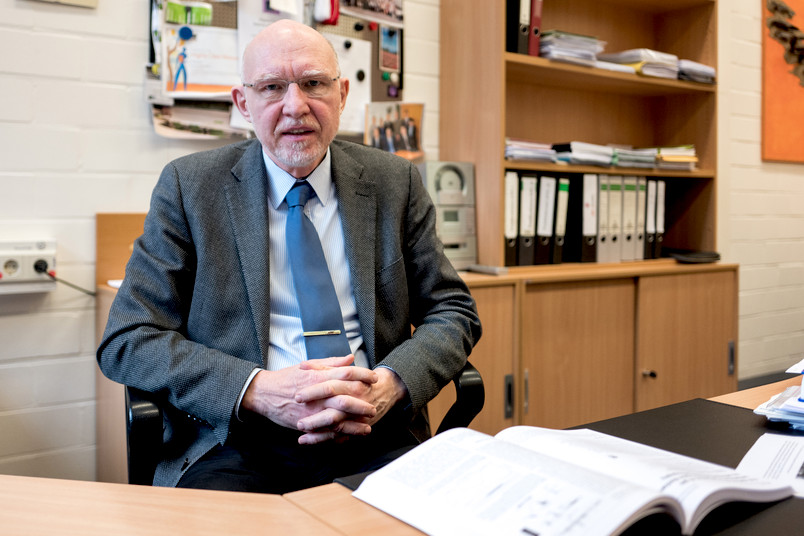